
Introduction to Anode vs. Cathode
Anodes and cathodes are essential in electrical and electrochemical systems, highlighting the core differences in anode vs. cathode functionality. The anode drives oxidation by releasing electrons, while the cathode enables reduction by accepting them. These opposing processes power everything from batteries to industrial systems, underscoring their importance in advancing modern technology.
What is an Anode?
The anode is the electrode where oxidation reactions occur, releasing electrons into the external circuit. In a galvanic cell, it acts as the negative electrode, while in an electrolytic cell, it functions as the positive electrode. Its primary role is to facilitate electron flow, enabling electrochemical reactions essential for energy generation or driving chemical processes efficiently.
Key Components of an Anode
- Conductive Matrix: The anode features a three-dimensional conductive structure, such as carbon matrices or metal foams. These provide a large surface area for reactions and support the deposition of active materials.
- Active Materials: Active materials directly participate in electrochemical reactions. Examples include lithium, zinc, aluminum, and compounds like silicon oxides or mixed metal hydrides, chosen for their high reactivity and energy density.
- Chemical Bonding Groups: Functional groups on the conductive matrix enhance bonding with active metals, ensuring uniform deposition and reducing uneven deposits or isolated clusters.
- Coatings and Additives: To improve performance, anodes are often coated with materials like tin, bismuth, or mercury. These coatings enhance electrochemical properties, boost cycle life, and minimize impedance, optimizing overall efficiency.
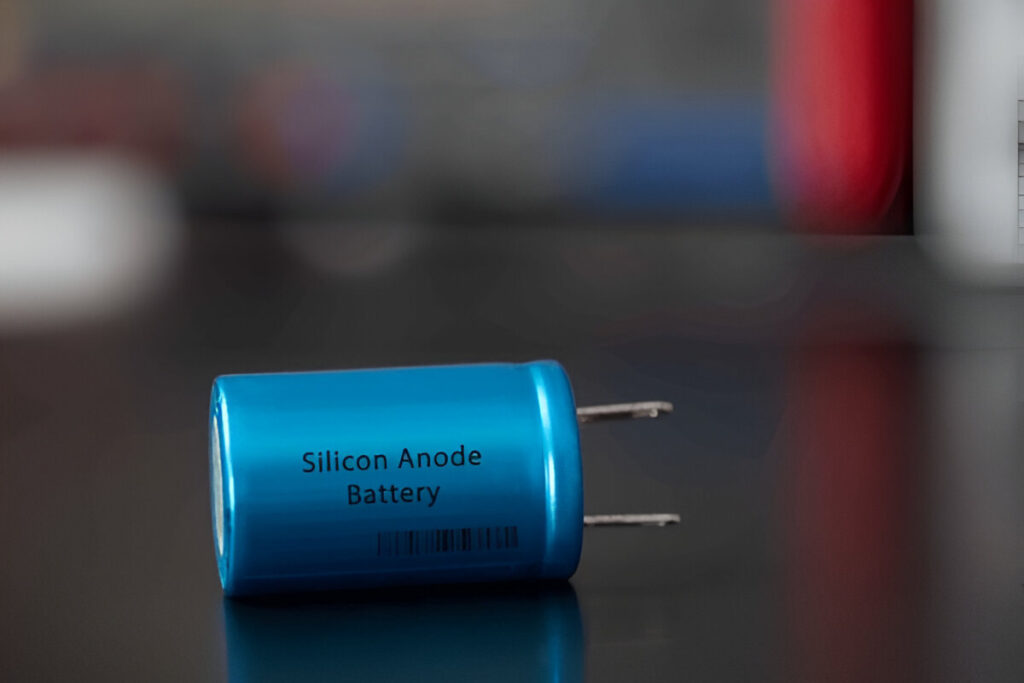
What is a Cathode?
The cathode is critical in electrochemical devices like batteries, fuel cells, supercapacitors, and electrochromic systems, directly influencing their performance and efficiency. Its primary role is enabling reduction reactions essential for energy storage and conversion.
Components of a Cathode
- Active Material: Cathode materials drive electrochemical reactions. Common options include lithium cobalt oxide (LiCoO2), lithium nickel oxide (LiNiO2), and lithium manganese oxide (LiMn2O4). These materials enable reversible lithium-ion intercalation, essential for lithium-ion batteries. Advanced materials like Li(Ni1Co)O2 and LiNi0.5Mn1.5O4 offer higher capacities and improved stability.
- Conductive Material: Conductive materials, such as carbon black, graphite, or polymers, enhance electron transport within the cathode. This ensures efficient electrochemical reactions and boosts overall device performance.
- Binder: Binders like polyvinylidene fluoride (PVDF) and styrene-butadiene rubber (SBR) hold active and conductive materials together. They provide mechanical stability and maintain cathode integrity during charge-discharge cycles.
Anode vs. Cathode: Key Differences
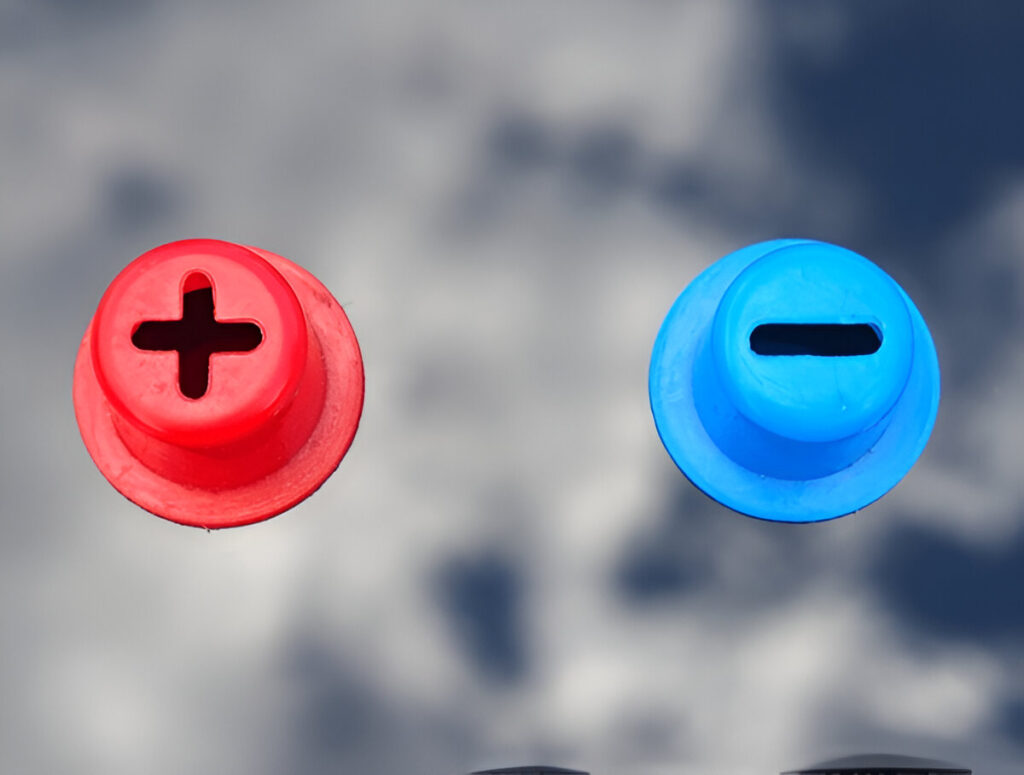
1. Fundamental Functional Differences
- Anode: The anode loses electrons during oxidation. In a discharging battery, it serves as the negative electrode, while in charging, it becomes positive. For example, lithium-ion battery anodes often use graphite, which undergoes oxidation to release electrons.
- Cathode: The cathode gains electrons during reduction. During discharge, it acts as the positive electrode, reversing to negative during charging. Common materials include lithium cobalt oxide (LiCoO2) in lithium-ion batteries and manganese dioxide (MnO2) in alkaline batteries.
2. Electrochemical Reactions
- Anode Reactions: Oxidation at the anode releases electrons into the circuit. For instance, in lithium-ion batteries, lithium ions intercalate into graphite, represented as:
Li→Li++e−\text{Li} \rightarrow \text{Li}^+ + e^-Li→Li++e− - Cathode Reactions: Reduction at the cathode involves electrons combining with ions. For lithium-ion batteries:
Li++e−+CoO2→LiCoO2\text{Li}^+ + e^- + \text{CoO}_2 \rightarrow \text{LiCoO}_2Li++e−+CoO2→LiCoO2
This reaction drives current flow in the external circuit.
3. Material Choices and Impact
- Anode Materials: Graphite is widely used for its stability and lithium-ion intercalation ability. Alternatives like silicon offer higher capacities but face challenges like volume expansion. Lithium titanate (Li4Ti5O12) provides stability and safety but has lower capacity.
- Cathode Materials: Lithium cobalt oxide (LiCoO2) offers high energy density, while lithium iron phosphate (LiFePO4) provides better safety and thermal stability. Material selection impacts battery voltage, energy capacity, and performance.
4. Electrochemical Mechanisms
- Ion Movement: During discharge, ions migrate from the anode to the cathode through the electrolyte, maintaining charge balance. Electrolytes must enable ion movement while preventing electron flow.
- Reversibility: High-quality electrode materials support reversible reactions, allowing repeated charge/discharge cycles without major degradation, ensuring efficiency.
- Aging and Degradation: Over time, issues like SEI layer formation and structural changes degrade electrodes. Effective battery management systems help reduce these effects and extend battery life.
5. Applications and Implications
- Energy Storage: Anode and cathode materials significantly influence the efficiency, capacity, and safety of systems like EV batteries, portable devices, and grid storage.
- Environmental Impact: Selecting non-toxic, recyclable materials for electrodes reduces the environmental footprint of battery production and disposal, supporting sustainability.
Application Cases
Product/Project | Technical Outcomes | Application Scenarios |
---|---|---|
Energy Storage Device | Reduces internal electron conductivity of particles, minimizing electrolyte consumption and degradation. | Electrochemical generators and cell components requiring efficient energy storage. |
Electrochemical Cell | Improves production of catalytic electrodes, meeting manufacturing process requirements. | Fuel and primary cell manufacturing, requiring efficient catalytic electrode production. |
Electrolytic System for CO2 Reduction | Enhances CO2-to-ethylene conversion via molecular tuning, promoting efficient electrochemical reduction. | Electrochemical systems for CO2 reduction, focusing on sustainable fuel production. |
Bioelectrochemical System | Produces methanol or derivatives from methane using bioanode and cathode, improving oxidation and reactive oxygen species production. | Bioelectrochemical systems for methanol production from methane, enhancing renewable energy applications. |
Electrochemical Cell | Prevents direct contact between anode and cathode, ensuring efficient ion transport and reducing risks of short circuits. | Medical devices and energy storage systems requiring reliable and safe electrochemical cells. |
FAQ
Why is the anode negative in a galvanic cell?
- In a galvanic cell, the anode is negative because it is the source of electrons that flow through the external circuit to the cathode, which is positive
Can the anode and cathode switch roles?
- Yes, the roles of anode and cathode can switch depending on the device’s operation. For example, during battery discharge, the anode is negative; during charging, it becomes positive.
How are anode and cathode identified in diodes?
- In diodes, the anode is the positive side, and the cathode is the negative side. Current flows from the anode to the cathode when the diode is forward-biased.
How do anode and cathode function in a battery?
- In a battery, the anode undergoes oxidation, releasing electrons, while the cathode undergoes reduction, accepting electrons. This electron flow generates electric current.
To get detailed scientific explanations of Anode vs. Cathode, try Patsnap Eureka.
