
Artificial Heart Evolution and Objectives
The artificial heart has been a subject of intense research and development for over six decades, driven by the growing need for alternative solutions to heart transplantation. The evolution of this technology can be traced back to the 1950s when the first mechanical heart pumps were developed. Since then, significant advancements have been made in materials science, bioengineering, and miniaturization, leading to more sophisticated and reliable artificial heart devices.
If you’re exploring the latest in artificial heart technology or need expert insights into its engineering and biomedical aspects, Eureka Technical Q&A offers quick, reliable answers from real specialists—making complex topics easier to understand and apply in real-world innovations.
The primary objective of artificial heart technology is to provide a viable long-term solution for patients with end-stage heart failure who are not eligible for heart transplantation or as a bridge to transplantation. This technology aims to replicate the function of the natural heart, pumping blood throughout the body and maintaining adequate circulation to support vital organ function. As the field progresses, researchers are focusing on developing devices that not only sustain life but also improve the quality of life for patients.
The evolution of artificial heart technology has been driven by the persistent need to address the global shortage of donor hearts and the limitations of current ventricular assist devices. The BiVacor TAH aims to overcome these challenges by offering a fully implantable, durable, and potentially permanent solution. Its development is rooted in the convergence of advanced materials science, miniaturized electronics, and sophisticated fluid dynamics modeling.
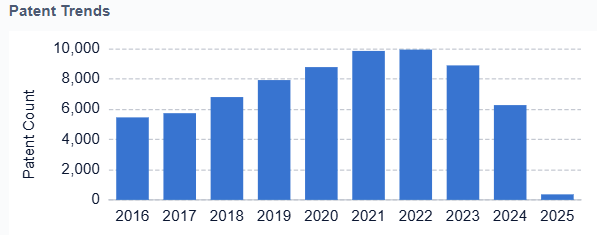
Key milestones in the evolution of artificial hearts include the first successful implantation of a total artificial heart in 1982, the development of continuous-flow devices in the 1990s, and the introduction of fully implantable systems in the 2000s. Each of these advancements has brought us closer to the ultimate goal of creating a permanent, reliable replacement for the human heart.
Current objectives in artificial heart technology include improving device durability and longevity, reducing the risk of complications such as thrombosis and infection, and developing more compact and energy-efficient systems. Researchers are also exploring the integration of smart technologies and biocompatible materials to enhance device performance and patient outcomes.
Another important aspect of artificial heart evolution is the development of partial support devices, such as ventricular assist devices (VADs), which can be used to support failing hearts without completely replacing them. These devices have shown promise in improving patient survival rates and quality of life, and their continued development is a key focus in the field.
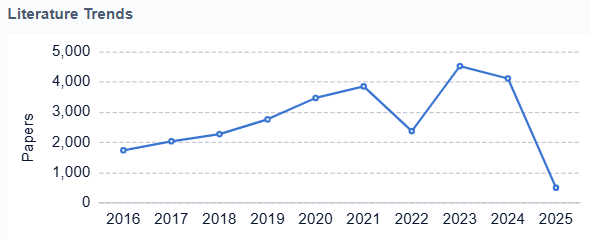
Looking ahead, the future of artificial heart technology is likely to involve advancements in biocompatible materials, improved power sources, and the integration of artificial intelligence for real-time monitoring and adjustment of device function. The ultimate goal remains the creation of a fully implantable, self-regulating artificial heart that can provide long-term support with minimal complications and maintenance requirements.
Artificial Heart Market Demand Analysis
The artificial heart market is growing rapidly and shows no signs of slowing down. Several key factors are fueling this expansion. These include rising global cases of cardiovascular disease, an aging population, and a shortage of donor hearts. According to market research, the global artificial heart market will reach $2.2 billion by 2027. It’s projected to grow at a compound annual growth rate (CAGR) of 10.5% from 2020 to 2027.
Demand is especially strong in countries with advanced healthcare systems. The United States, Germany, and Japan lead the way in adoption. These countries face high rates of heart failure and offer the infrastructure for complex surgeries. Meanwhile, emerging markets like China and India are catching up. Improving healthcare access and rising incomes are driving demand in these regions.
One major factor behind this growth is the rising number of end-stage heart failure patients. Over 26 million people worldwide live with heart failure. In the United States alone, more than 500,000 new cases are diagnosed every year. As a result, the need for alternative treatments is growing. Artificial hearts give new hope to patients who can’t receive transplants or are waiting for donor hearts.
Technological innovation is another key driver of market expansion. Modern artificial hearts now offer better durability and fewer complications. Their improved performance makes them more suitable for long-term care. As a result, doctors and patients see them as more than just a last resort.
Another trend shaping the market is the move toward fully implantable artificial hearts. Today, most devices like the SynCardia Total Artificial Heart need external components. However, new systems aim to be fully self-contained. These advances promise greater mobility and better quality of life for patients.
The COVID-19 pandemic disrupted production and delayed many elective surgeries. Yet, it also highlighted the importance of medical innovation. In the long run, the pandemic may speed up the adoption of life-saving technologies like artificial hearts.
Despite strong momentum, the market faces real challenges. High production and surgical costs still limit access in many areas. Complex regulations and the need for expert surgeons also slow adoption. In addition, research in regenerative medicine and stem cells could offer new alternatives in the future.
In conclusion, the artificial heart market offers major growth potential. Rising disease rates, strong innovation, and urgent medical needs support expansion. However, to unlock its full value, companies must address cost, regulation, and competition. Only then can they deliver solutions that are both effective and accessible.
Current Artificial Heart Technology Landscape
The current artificial heart technology landscape is characterized by significant advancements in both total artificial hearts (TAHs) and ventricular assist devices (VADs). TAHs, designed to completely replace the native heart, have evolved from pneumatically driven systems to more compact, electrically powered devices. The SynCardia temporary Total Artificial Heart remains the only FDA-approved TAH, capable of supporting patients for extended periods while awaiting transplantation. Meanwhile, VADs, which assist rather than replace the heart, have seen remarkable progress in miniaturization and durability. The latest generation of continuous-flow VADs, such as the HeartMate 3 and HVAD, offer improved outcomes and reduced complications compared to earlier pulsatile models.
Technological innovations have focused on addressing key challenges in artificial heart development, including thrombosis, infection, and device durability. Researchers have introduced advanced materials and surface modifications to improve biocompatibility and reduce blood clot risks. They are also developing transcutaneous energy transfer systems to prevent driveline infections, a major issue with current devices. Additionally, efforts are underway to create fully implantable artificial hearts with integrated power sources, potentially improving patient quality of life and reducing infection risks.
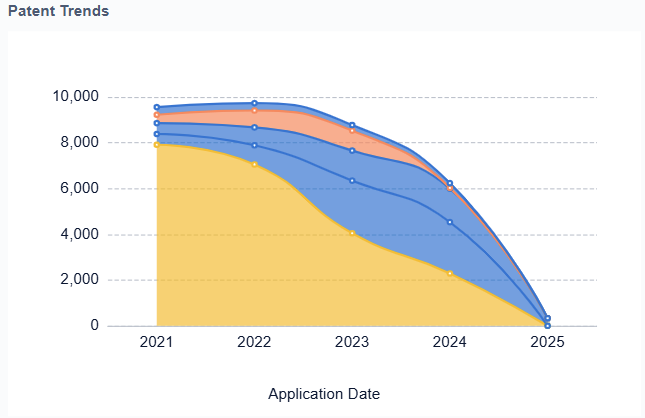
The field has also witnessed the emergence of novel designs, such as the soft robotic heart developed by researchers at Harvard University and Boston Children’s Hospital. This approach mimics the natural movement of the heart muscle, potentially offering a more physiological solution. Another promising development is the use of 3D printing technology to create custom-fitted artificial hearts, addressing the challenge of size mismatch in pediatric patients.
Despite these advancements, several technical challenges persist. Power management remains a critical issue, with researchers exploring more efficient batteries and wireless charging technologies. Achieving a balance between device size and functional capacity continues to be a focus area, particularly for pediatric applications. Additionally, the development of smart artificial hearts with integrated sensors and adaptive control systems is an emerging trend, aiming to optimize device performance based on patient activity levels and physiological demands.
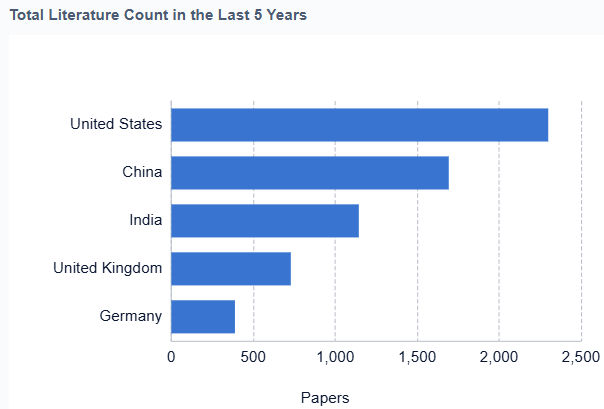
The regulatory landscape for artificial heart technology is evolving, with agencies like the FDA working to streamline approval processes while maintaining rigorous safety standards. This has led to increased collaboration between academic institutions, medical device companies, and regulatory bodies to accelerate innovation in the field. As the technology continues to advance, there is a growing emphasis on long-term studies to assess the durability and efficacy of these devices, particularly as bridge-to-transplant solutions evolve into destination therapy options for select patient populations.
Artificial Heart Development Timeline
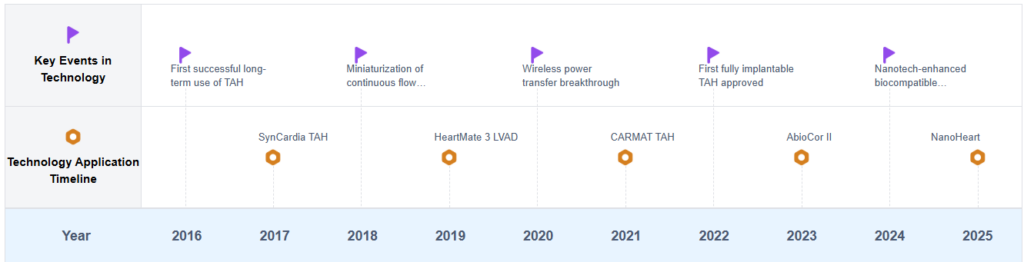
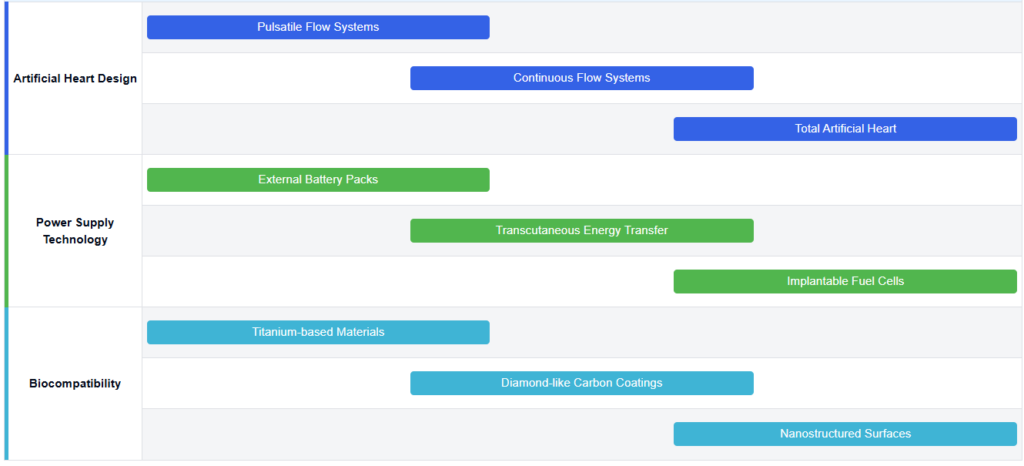
Key Players in Artificial Heart Industry
The artificial heart market is in a growth phase, driven by increasing prevalence of heart failure and advancements in technology. The global market size is projected to expand significantly in the coming years. Technical maturity varies across different aspects of artificial heart technology. Companies like SynCardia Systems LLC and Berlin Heart GmbH are leading in total artificial heart development, while firms such as Medtronic and Abbott are advancing ventricular assist devices. Universities and research institutions, including the University of Cincinnati, Beijing University of Technology, and the University of California, are contributing to innovation in this field. Key challenges include improving device durability, reducing complications, and miniaturizing components. Future prospects are promising, with ongoing research focusing on biocompatible materials, energy transfer systems, and smart control mechanisms.
magAssist Co., Ltd.
Technical Solution
magAssist Co., Ltd. has developed a novel magnetic levitation artificial heart pump. Their technology utilizes magnetic levitation to suspend the rotor, eliminating mechanical contact and reducing wear and tear. The pump is designed to be fully implantable and provides continuous flow support for patients with advanced heart failure. magAssist’s device incorporates advanced control algorithms to adjust pump speed based on the patient’s physiological needs, mimicking the natural heart’s response to varying demands. The company is also working on integrating wireless power transfer technology to eliminate the need for percutaneous drivelines, reducing infection risks.
Strengths: Magnetic levitation technology reduces mechanical wear, potentially increasing device longevity. Advanced control algorithms allow for physiological responsiveness.
Weaknesses: Limited long-term clinical data available. Continuous flow design may not fully replicate pulsatile flow of natural heart.
Shanghai NewMed Medical Co., Ltd.
Technical Solution
Shanghai NewMed Medical Co., Ltd. has developed an innovative left ventricular assist device (LVAD) called HeartCon. This device is designed to be a long-term solution for patients with end-stage heart failure. The HeartCon LVAD uses a magnetically levitated centrifugal pump that provides continuous blood flow support. It features a compact design that allows for minimally invasive implantation procedures. The device incorporates advanced sensor technology to monitor pump performance and patient hemodynamics, enabling real-time adjustments and optimized therapy. NewMed is also working on developing a fully implantable system with transcutaneous energy transfer to eliminate driveline-related complications.
Strengths: Compact design allows for less invasive implantation. Advanced monitoring capabilities enable personalized treatment.
Weaknesses: Limited global market presence compared to established Western competitors. Long-term clinical data still being accumulated.
SynCardia Systems LLC
Technical Solution
SynCardia Systems LLC has developed the world’s first and only FDA-approved Total Artificial Heart (TAH). Their technology, the SynCardia temporary Total Artificial Heart, is designed to replace the failing heart’s two ventricles and four valves. It pumps up to 9.5 liters per minute through both ventricles to provide enhanced blood flow to vital organs. The device is used as a bridge to transplant for patients at risk of imminent death from biventricular failure. SynCardia’s TAH is pneumatically driven and powered by an external driver, allowing for patient mobility and hospital discharge while waiting for a donor heart.
Strengths: FDA-approved, proven technology with over 1,800 implants worldwide. Provides full circulatory support, eliminating symptoms of end-stage biventricular failure.
Weaknesses: External power source limits patient mobility, risk of infection at driveline exit site, and the need for anticoagulation therapy.
Massachusetts Institute of Technology
Technical Solution
MIT has been at the forefront of artificial heart research, focusing on developing more compact and efficient devices. Their approach involves creating a soft robotic heart that mimics the structure and function of the natural heart. This artificial heart uses soft, flexible materials and is powered by compressed air. The device consists of an actuated double-sided silicone membrane that separates two chambers, replicating the pumping action of the heart. MIT’s design aims to reduce the risk of blood clots and improve overall biocompatibility compared to traditional mechanical artificial hearts.
Strengths: Innovative soft robotic design potentially reduces blood clotting risks and improves biocompatibility. The use of soft materials may lead to less damage to blood cells.
Weaknesses: Still in early stages of development, not yet ready for human trials. Long-term durability of soft materials in a high-stress environment needs to be proven.
Current Artificial Heart Design Solutions
Design and structure of artificial heartsArtificial hearts are designed to mimic the function of natural hearts, with various structural innovations to improve efficiency and durability. These designs may include features such as multiple chambers, specialized valves, and advanced pumping mechanisms to ensure optimal blood flow and reduce the risk of complications.
- Pump-based artificial heart designs
Artificial hearts often utilize pump-based designs to mimic the function of the natural heart. These designs may include continuous flow pumps or pulsatile pumps that circulate blood through the body. The pumps can be electrically powered and controlled to adjust blood flow based on the patient’s needs. Some designs incorporate multiple chambers or ventricles to more closely replicate natural heart function. - Materials and biocompatibility
The choice of materials is crucial in artificial heart design to ensure biocompatibility and durability. Engineers often use advanced materials like titanium alloys, polyurethane, and carbon-based composites in artificial heart components. They choose these materials for their strength, durability, and ability to reduce blood clotting. They also apply specialized coatings and surface treatments to improve hemocompatibility and lower infection risks. - Control systems and power supply
Artificial hearts incorporate sophisticated control systems to regulate blood flow and respond to changing physiological demands. These systems may use sensors to monitor various parameters such as blood pressure, oxygen levels, and activity levels. Power supply is a critical aspect, with designs featuring rechargeable batteries, transcutaneous energy transfer systems, or even implantable fuel cells to ensure continuous operation. - Implantation techniques and anatomical fit
The design of artificial hearts must consider implantation techniques and anatomical fit within the chest cavity. Some designs aim to be fully implantable, while others may have external components. Considerations include minimizing the size of the device, creating appropriate connections to the patient’s vasculature, and ensuring compatibility with surgical procedures. Some designs may be customized to fit individual patient anatomy using advanced imaging and 3D printing technologies.
Power systems for artificial hearts
Artificial hearts require reliable power sources to function continuously. Innovations in this area include advanced battery technologies, wireless power transmission systems, and energy-efficient motors. These developments aim to extend the operational life of artificial hearts and improve patient mobility.
Control and monitoring systems
Sophisticated control and monitoring systems are crucial for the proper functioning of artificial hearts. These systems may incorporate sensors, microprocessors, and wireless communication technologies to adjust heart rate, monitor blood flow, and detect potential issues. Advanced algorithms and artificial intelligence may be used to optimize performance and predict maintenance needs.
Biocompatibility and integration
Improving the biocompatibility of artificial hearts is essential for reducing rejection and complications. This includes developing new materials that are less likely to cause blood clots or immune responses, as well as designing surfaces that promote better integration with the patient’s tissues. Some approaches may involve coating the devices with bioactive substances or using tissue engineering techniques.
Miniaturization and implantation techniques
Efforts to miniaturize artificial hearts and improve implantation techniques aim to make the devices suitable for a wider range of patients, including children and smaller adults. This involves developing compact designs, minimally invasive surgical procedures, and innovative anchoring methods to ensure proper placement and function within the chest cavity.
Core Innovations in Artificial Heart Technology
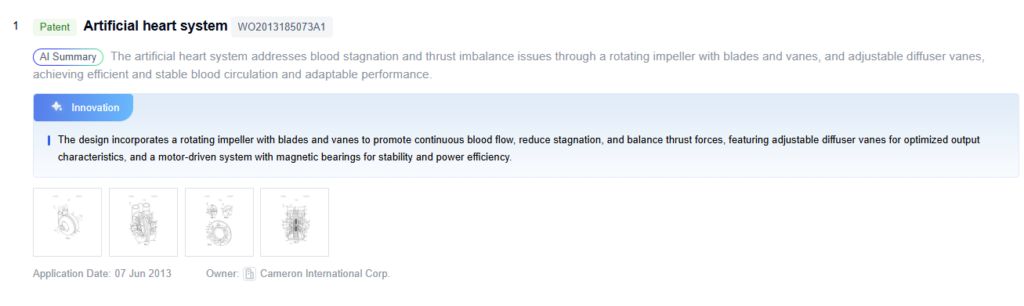
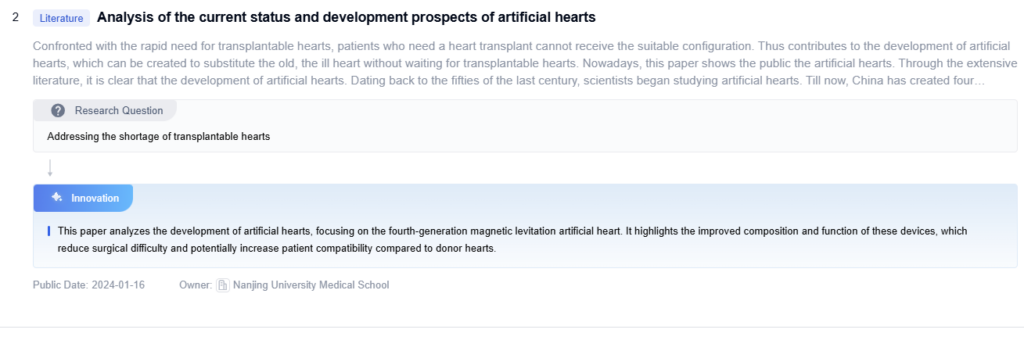
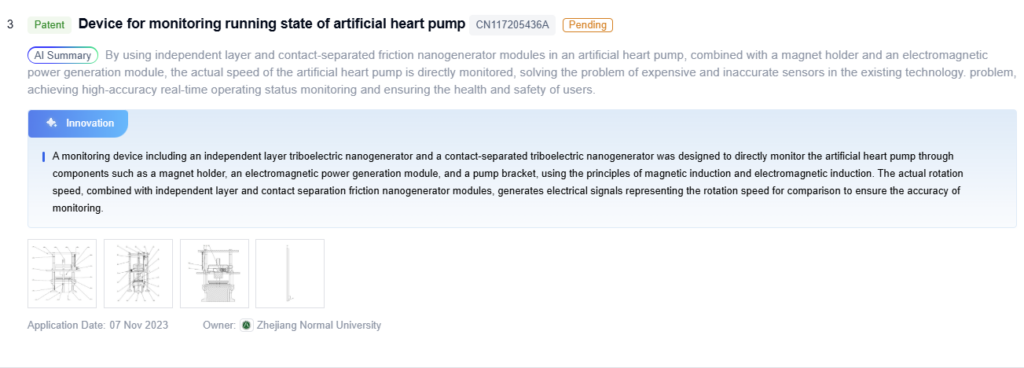
Future Artificial Heart Research Directions
Energy Harvesting and Wireless Power Transfer in Artificial Hearts
The future of artificial heart technology is moving toward energy independence. Researchers are developing advanced energy harvesting and wireless power transfer systems to eliminate external power sources and transcutaneous power cables, which are common in current devices.
Harnessing the Body’s Natural Energy
Scientists are exploring how to convert the body’s natural energy into electricity. One method uses thermoelectric generators, which transform the temperature difference between the body’s core and skin into power. Another uses piezoelectric materials to generate electricity from natural movements like breathing or heartbeat. Researchers are also testing triboelectric nanogenerators that harvest energy from friction between materials within the body.
Advances in Wireless Power Transfer
Parallel efforts focus on wireless energy delivery. Resonant inductive coupling systems are becoming more efficient at transmitting power through biological tissue. Ultrasonic power transfer is also gaining attention due to its deeper penetration and higher efficiency compared to electromagnetic methods. These advances could allow continuous energy delivery without external connections.
Optimizing Power Management
To make the most of harvested or wirelessly transferred energy, engineers are designing miniaturized power management circuits. These systems stabilize variable inputs and ensure reliable power for the artificial heart. They are also working on integrating thin-film batteries and supercapacitors to store energy and provide backup during low-power periods.
Exploring Biochemical Energy Sources
Some researchers are investigating how the body’s own chemistry could generate power. Concepts like glucose oxidation could create bioelectricity, though this technology is still in its early stages and faces challenges with stability and energy output.
Strengths of Energy Harvesting and Wireless Power Systems
- Removes the need for external cables, lowering infection risks
- Increases patient mobility and comfort
- Reduces the need for battery replacement surgeries
- Opens the door for self-sustaining artificial hearts
Challenges to Overcome
- Current harvesting methods may not produce enough power
- Maintaining stable energy output is difficult under varying conditions
- Long-term safety of wireless energy fields remains under review
Regulatory Framework for Artificial Hearts
Biocompatibility and long-term durability are two of the most critical challenges in the advancement of artificial heart technology. Because the human body naturally rejects foreign materials, artificial hearts must use components that can remain compatible with biological tissues over extended periods. Current designs typically rely on titanium alloys and polyurethane for structural and blood-contacting surfaces. While these materials offer good strength and relative compatibility, they still face issues when it comes to maintaining long-term performance inside the human body.
One of the major concerns is the risk of blood clot formation on the surface of the artificial heart. Clots can lead to dangerous complications such as thromboembolism or device failure. Researchers are working on various solutions, including applying heparin coatings or using endothelialization techniques that mimic natural blood vessels. Although these methods have shown promise in reducing thrombosis, maintaining their effectiveness over many years is still a major hurdle.
Mechanical components within the artificial heart, particularly in continuous-flow designs, present additional challenges. Constant rotation of impellers and bearings can result in material fatigue and the release of microscopic particles into the bloodstream. These mechanical issues compromise device function and patient safety. To address this, engineers are developing magnetic levitation systems that reduce physical wear and improve long-term reliability. However, these newer systems also require extensive validation through years of testing.
Another important issue is the body’s reaction to implanted devices. Inflammation and fibrotic tissue growth around the implant site can interfere with device function. To minimize these responses, researchers are exploring bioactive materials and surface treatments that encourage natural tissue integration and reduce adverse reactions. These improvements aim to make the device more compatible with surrounding tissues and enhance patient outcomes.
Power supply systems also need to meet durability expectations. Transcutaneous energy transfer systems (TETS) and implantable batteries must function reliably for many years inside the body. These systems face threats such as corrosion, inefficiency, and degradation over time. Any failure in power delivery can lead to life-threatening complications, which underscores the need for robust, long-lasting energy solutions within the artificial heart system.
Long-term durability testing is another complex challenge. While in vitro studies and accelerated aging tests help estimate performance, they can’t fully replicate the biological conditions inside a human body. Likewise, long-term animal studies provide valuable data but still fall short of capturing the full range of variables that can influence device performance over a decade or more of use.
To overcome these challenges, scientists and engineers are taking a multidisciplinary approach. Researchers are developing novel biomaterials that respond dynamically to the biological environment and remain stable over time. Some are exploring self-healing materials that can automatically repair minor wear and damage. Others are building more sophisticated computer models to simulate the real-world conditions an artificial heart will face inside the body. These innovations aim to enhance both the biocompatibility and the mechanical resilience of future devices.
Ultimately, addressing the issues of biocompatibility and long-term durability will determine the success of artificial hearts as long-term or permanent solutions for patients with end-stage heart failure. Continued advancements in materials science, bioengineering, and implantable power systems will be essential to ensure that artificial hearts can perform reliably for years, improving patient outcomes and quality of life.
To get detailed scientific explanations of Artificial Heart, try Patsnap Eureka.
