
Borophene Background and Research Objectives
Borophene, a two-dimensional allotrope of boron, has emerged as a promising material in the field of nanotechnology. Since its theoretical prediction in the 1990s and subsequent experimental synthesis in 2015, borophene has garnered significant attention from researchers and industry experts alike. This atomically thin material exhibits unique properties that set it apart from other 2D materials, such as graphene, making it a subject of intense study and exploration.
Curious about borophene’s future in industry? Eureka Technical Q&A offers expert insights into borophene research, its industrial applications in energy storage, electronics, and nanotechnology, and its market growth potential in advanced material innovation.
The development of borophene can be traced back to the broader context of 2D materials research, which gained momentum following the isolation of graphene in 2004. As scientists sought to expand the family of 2D materials, boron emerged as a candidate due to its electron-deficient nature and ability to form diverse bonding configurations. The journey from theoretical prediction to experimental realization spanned over two decades, highlighting the challenges involved in synthesizing and characterizing this novel material.
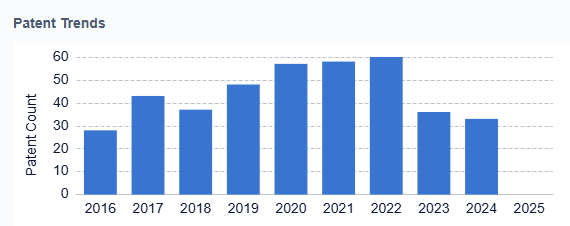
The primary objectives of borophene research are multifaceted. Firstly, researchers aim to develop reliable and scalable synthesis methods to produce high-quality borophene sheets. Current techniques, such as molecular beam epitaxy, are limited in their scalability, presenting a significant hurdle for industrial applications. Secondly, there is a pressing need to fully characterize the material’s properties, including its mechanical strength, electrical conductivity, and chemical reactivity. Understanding these properties is crucial for identifying potential applications and optimizing the material for specific use cases.
Another key research objective is to explore borophene’s potential in various technological domains. Initial studies have suggested promising applications in energy storage, catalysis, and electronics. For instance, borophene’s high theoretical specific capacity makes it an attractive candidate for next-generation batteries, while its catalytic properties could revolutionize hydrogen evolution reactions. In the field of electronics, borophene’s anisotropic conductivity opens up possibilities for novel device architectures.
As research progresses, the focus is shifting towards addressing practical challenges that hinder borophene’s transition from laboratory curiosity to industrial reality. This includes developing methods to transfer borophene sheets from growth substrates, enhancing the material’s stability under ambient conditions, and scaling up production to meet potential industrial demands. Additionally, researchers are exploring ways to functionalize borophene and create heterostructures with other 2D materials, potentially unlocking new properties and applications.
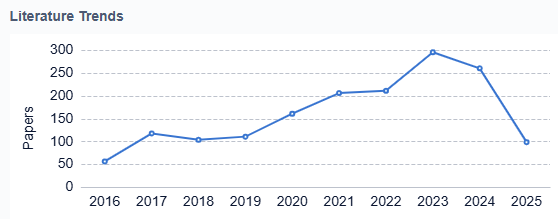
The ultimate goal of borophene research is to position this material as a key player in the next generation of technological innovations. By leveraging its unique properties, scientists and engineers hope to develop advanced materials and devices that outperform current technologies in areas such as energy storage, sensing, and nanoelectronics. As the field advances, interdisciplinary collaboration between materials scientists, chemists, physicists, and engineers will be crucial in realizing borophene’s full potential and driving its industrial applications forward.
Market Potential for Borophene-based Products
Borophene, a two-dimensional allotrope of boron, has emerged as a promising material with potential applications across various industries. The market potential for borophene-based products is significant, driven by its unique properties and versatility. As research and development in this field progress, several key market segments are expected to benefit from borophene integration.
In the electronics industry, borophene’s exceptional electrical conductivity and flexibility make it a strong candidate for next-generation flexible electronics and high-performance transistors. The global flexible electronics market is projected to reach $40 billion by 2025, with borophene potentially capturing a significant share. Additionally, borophene’s superconducting properties at room temperature could revolutionize energy transmission and storage systems, tapping into the growing renewable energy market, estimated to reach $1.5 trillion by 2025.
The aerospace and automotive sectors present another lucrative opportunity for borophene-based products. Its lightweight nature and superior strength-to-weight ratio make it ideal for developing advanced composite materials. These could be used in manufacturing lighter, more fuel-efficient vehicles and aircraft, addressing the increasing demand for sustainable transportation solutions. The global composite materials market, valued at $90 billion in 2020, is expected to grow at a CAGR of 7.5% until 2027, with borophene-enhanced composites potentially capturing a significant portion of this growth.
In the healthcare industry, borophene’s biocompatibility and unique surface properties make it a promising material for drug delivery systems and biosensors. The global drug delivery market is projected to reach $2.2 trillion by 2026, with novel materials like borophene driving innovation in targeted therapies and personalized medicine. Moreover, the biosensors market, expected to reach $36 billion by 2027, could see significant advancements through borophene-based sensing technologies.
The water treatment sector also presents a substantial market opportunity for borophene-based products. Its exceptional ability to remove salt and contaminants from water could address global water scarcity issues. The water treatment chemicals market, valued at $30 billion in 2020, is projected to grow at a CAGR of 6% until 2025, with borophene-based filtration systems potentially disrupting traditional treatment methods.
While the market potential for borophene-based products is promising, it’s important to note that commercialization challenges remain. These include scaling up production, ensuring cost-effectiveness, and addressing potential environmental and health concerns. As research progresses and these challenges are overcome, the market for borophene-based products is expected to grow significantly, potentially reaching billions of dollars across multiple industries within the next decade.
Borophene Synthesis Challenges and Limitations
Borophene, a two-dimensional allotrope of boron, has garnered significant attention in the scientific community due to its unique properties and potential applications. However, the synthesis of borophene presents several challenges and limitations that hinder its widespread industrial adoption. One of the primary obstacles is the difficulty in producing large-scale, high-quality borophene sheets. Current synthesis methods, such as molecular beam epitaxy and chemical vapor deposition, are limited to producing small-scale samples, typically on the order of micrometers. This size limitation restricts the ability to fully exploit borophene’s properties in practical applications and makes it challenging to integrate into existing manufacturing processes.
Another significant challenge is the inherent instability of borophene in ambient conditions. Unlike graphene, which is relatively stable in air, borophene is highly reactive and prone to oxidation. This instability necessitates the development of protective measures or encapsulation techniques to preserve the material’s integrity during handling and application. The high reactivity of borophene also complicates its characterization and analysis, as exposure to air or moisture can rapidly alter its properties.
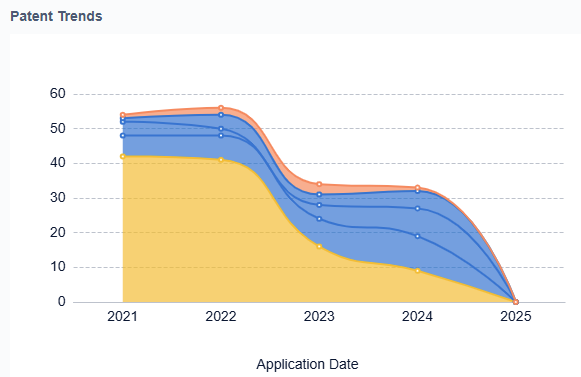
The choice of substrate for borophene growth is another critical factor that limits its synthesis. Currently, borophene has been successfully grown on a limited number of metallic substrates, primarily silver and gold. The strong interaction between borophene and these substrates affects the material’s electronic properties and makes it challenging to transfer the synthesized sheets to other substrates for device fabrication. This substrate dependency restricts the versatility of borophene in various applications and hinders its integration into existing technologies.
Furthermore, the precise control of borophene’s atomic structure during synthesis remains a significant challenge. Unlike graphene, which has a well-defined honeycomb structure, borophene can form various polymorphs with different atomic arrangements. These polymorphs exhibit distinct properties, and the ability to selectively produce specific structures is crucial for tailoring borophene’s characteristics to suit particular applications. However, current synthesis methods lack the level of control required to consistently produce desired polymorphs, limiting the reproducibility and scalability of borophene production.
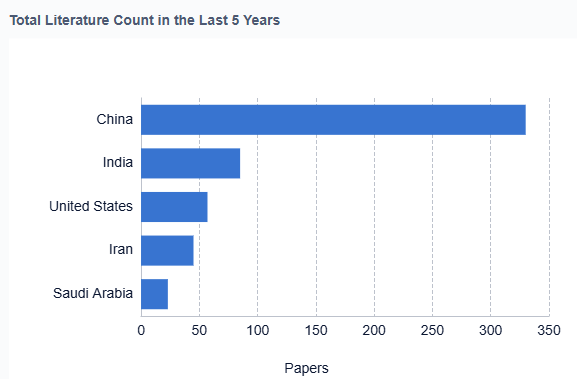
The high cost and complexity of current synthesis techniques also pose significant barriers to the industrial-scale production of borophene. The ultra-high vacuum conditions and specialized equipment required for molecular beam epitaxy, for instance, make the process expensive and difficult to scale up. Additionally, the low yield and time-consuming nature of current synthesis methods further contribute to the economic challenges of borophene production.
Addressing these synthesis challenges and limitations is crucial for realizing the full potential of borophene in industrial applications. Ongoing research efforts are focused on developing new synthesis methods, improving substrate compatibility, enhancing stability, and increasing production scale. Overcoming these hurdles will be essential for transitioning borophene from a laboratory curiosity to a commercially viable material with widespread industrial applications.
Borophene Development Timeline and Milestones
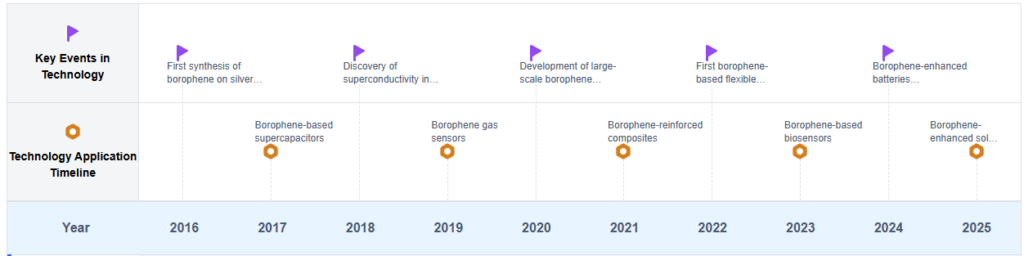
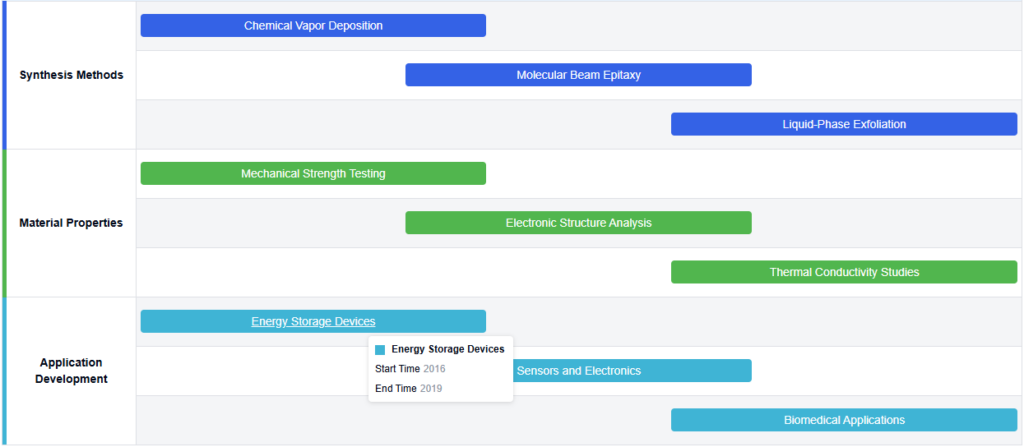
Key Players in Borophene Research and Development
The research on Borophene’s industrial applications and growth forecast is in its early stages, with the market still emerging. The technology’s maturity is relatively low, as evidenced by the involvement of primarily academic institutions like Northwestern University, Tsinghua University, and Tokyo Institute of Technology. However, interest from industry players such as BASF SE, Johnson Matthey Plc, and ExxonMobil Chemical Patents, Inc. suggests growing commercial potential. The competitive landscape is characterized by a mix of universities, research institutes, and corporations, indicating a collaborative approach to advancing Borophene technology. As the field progresses, we can expect increased market size and more diverse industrial applications, driven by ongoing research and development efforts.
Northwestern University
Technical Solution
Northwestern University has been at the forefront of borophene research since its initial synthesis in 2015. Their approach involves growing atomically thin sheets of boron on silver substrates under ultra-high vacuum conditions. They have successfully created various borophene structures, including β12 and χ3 phases, by controlling growth parameters. The university’s research focuses on understanding the fundamental properties of borophene and exploring its potential applications in electronics, energy storage, and catalysis.
Strengths: Pioneering research in borophene synthesis, extensive experience in characterization techniques.
Weaknesses: Limited scalability of current production methods, challenges in transferring borophene from growth substrates.
Institute of Process Engineering, Chinese Academy of Sciences
Technical Solution
The Institute of Process Engineering at the Chinese Academy of Sciences has developed innovative methods for large-scale production of borophene nanosheets. Their approach involves a liquid-phase exfoliation technique using organic solvents and ultrasonication to produce borophene from bulk boron. This method allows for the production of borophene nanosheets with controlled thickness and lateral dimensions. The institute is also exploring the functionalization of borophene for applications in energy storage, catalysis, and environmental remediation.
Strengths: Scalable production method, potential for industrial applications.
Weaknesses: Lower quality and purity compared to UHV-grown borophene, challenges in controlling sheet morphology.
BASF SE
Technical Solution
BASF SE, a global leader in chemical manufacturing, has been investing in borophene research for potential industrial applications. Their approach focuses on developing borophene-based composites and hybrid materials for use in catalysis, energy storage, and lightweight structural materials. BASF’s research includes the integration of borophene into polymer matrices and the development of borophene-based coatings for corrosion protection and thermal management. They are also exploring the use of borophene in next-generation battery technologies, particularly as an anode material for high-performance lithium-ion batteries.
Strengths: Strong industrial research capabilities, potential for rapid commercialization.
Weaknesses: Limited experience in fundamental borophene synthesis, reliance on external suppliers for raw materials.
Panjab University Chandigarh
Technical Solution
Panjab University Chandigarh has been conducting research on borophene’s applications in environmental remediation and water treatment. Their approach involves developing borophene-based adsorbents and membranes for the removal of heavy metals and organic pollutants from water. The university’s research team has successfully synthesized borophene-polymer composites with enhanced adsorption capacities for various contaminants. They are also investigating the use of borophene in photocatalytic water splitting for hydrogen production and as a support material for catalysts in wastewater treatment processes.
Strengths: Innovative applications in environmental technologies, focus on practical solutions. Weaknesses: Limited resources for large-scale production, challenges in long-term stability of borophene-based materials in aqueous environments.
Tsinghua University
Technical Solution
Tsinghua University has been conducting cutting-edge research on borophene’s electronic and optoelectronic properties. Their approach involves theoretical modeling and experimental validation of borophene’s unique electronic structure and its potential in nanoelectronics. The university’s research team has developed methods for creating borophene-based field-effect transistors and exploring its applications in flexible electronics. They are also investigating borophene’s potential in quantum computing, particularly its use as a platform for topological quantum bits. Additionally, Tsinghua researchers are exploring borophene’s thermoelectric properties for energy harvesting applications.
Strengths: Strong theoretical foundation, advanced characterization techniques.
Weaknesses: Challenges in device fabrication and integration, limited industrial partnerships for commercialization.
Current Borophene Synthesis and Application Methods
Synthesis and production of boropheneVarious methods for synthesizing and producing borophene, a two-dimensional allotrope of boron, are described. These techniques may involve chemical vapor deposition, molecular beam epitaxy, or other advanced manufacturing processes to create single-layer or few-layer borophene structures.
- Synthesis methods for borophene
Various techniques are employed for synthesizing borophene, including chemical vapor deposition, molecular beam epitaxy, and liquid-phase exfoliation. These methods aim to produce high-quality, large-area borophene sheets with controlled thickness and structure. - Substrate selection and preparation
The choice and preparation of substrates play a crucial role in borophene synthesis. Metallic substrates like silver, gold, or copper are commonly used. Surface treatment and atomically flat preparation of these substrates are essential for successful borophene growth. - Post-synthesis processing and characterization
After synthesis, borophene undergoes various post-processing steps and characterization techniques. These may include transfer processes to different substrates, surface functionalization, and advanced microscopy and spectroscopy methods to analyze the structure and properties of the produced borophene. - Scale-up and industrial production considerations
Scaling up borophene production for industrial applications presents challenges. Research focuses on developing continuous production methods, optimizing growth parameters for large-area synthesis, and addressing issues related to uniformity and defect control in large-scale production.
Applications of borophene in electronic devices
Borophene’s unique electronic properties make it suitable for various applications in electronic devices. These may include use in transistors, sensors, supercapacitors, and other nanoelectronic components, leveraging its high conductivity and tunable band gap.Expand
Borophene-based energy storage and conversion
The potential of borophene in energy storage and conversion applications is explored. This includes its use in batteries, fuel cells, and photovoltaic devices, taking advantage of its high surface area and electron mobility.Expand
Functionalization and modification of borophene
Methods for functionalizing and modifying borophene to enhance its properties or tailor it for specific applications are discussed. This may involve chemical treatments, doping, or creating hybrid structures with other materials.Expand
Borophene in composite materials and coatings
The incorporation of borophene into composite materials and coatings is explored. This includes its use to enhance mechanical, thermal, or electrical properties of materials, as well as potential applications in protective or functional coatings.
Breakthrough Patents in Borophene Technology
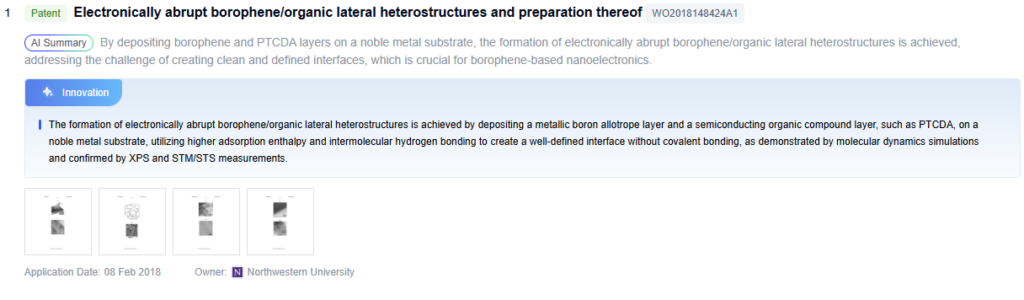
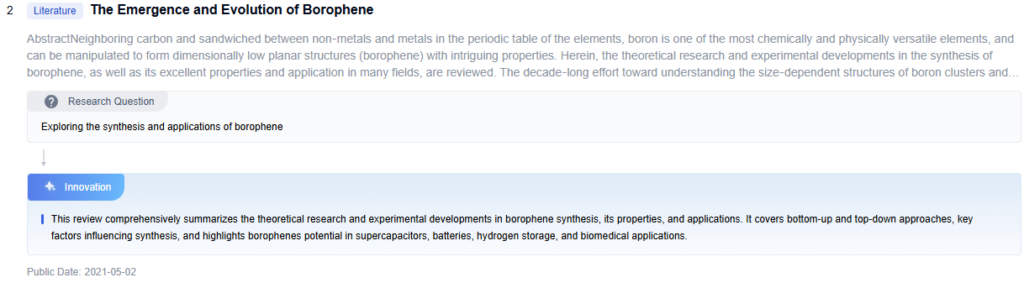
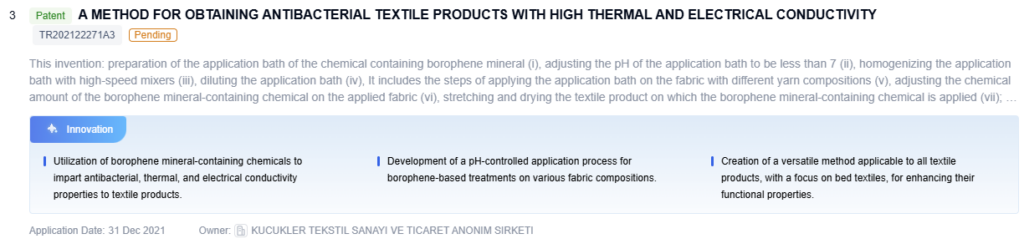
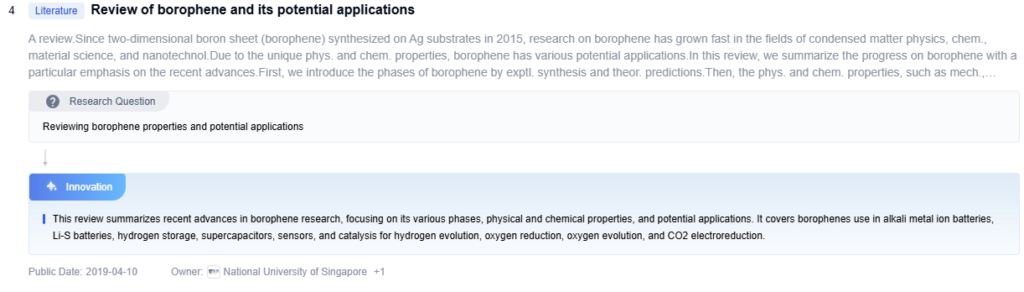
Future Directions in Borophene Applications
Borophene-Based Flexible Electronics
Borophene, a two-dimensional form of boron, is emerging as a breakthrough material for flexible electronics. Its extraordinary electrical conductivity, mechanical flexibility, and atomic thinness make it highly attractive for next-generation devices.
Researchers are focusing on synthesizing large-area borophene sheets using advanced techniques like molecular beam epitaxy or chemical vapor deposition. They transfer these sheets onto flexible substrates such as polyimide or PET to create highly adaptable electronic devices.
They are also developing borophene-based transistors, sensors, and interconnects designed specifically for flexibility and performance. Engineers are improving borophene synthesis to produce larger, defect-free sheets for better device performance.
In addition, scientists are exploring methods to maintain borophene’s integrity during the transfer process. Designing flexible transistors with high carrier mobility and developing stretchable circuits that self-heal after damage are key goals.
Researchers aim to integrate borophene with other 2D materials to create heterostructures that offer enhanced functionality. This integration can lead to improved performance in a variety of devices.
Borophene-based flexible electronics show great potential in wearable health monitors, foldable displays, soft robotics, and smart textiles. They may also play a role in flexible energy storage devices, including supercapacitors and batteries, due to their exceptional conductivity and flexibility.
However, several challenges remain. Scaling up borophene production is difficult, and its sensitivity to air and moisture limits its stability. Additionally, integrating borophene into complex systems requires new manufacturing methods.
To overcome these hurdles, collaboration between material scientists, electrical engineers, and device manufacturers is essential. These partnerships will help bring borophene-based flexible electronics closer to commercial applications.
Strengths of Borophene Flexible Electronics
Borophene provides unmatched flexibility, excellent electrical conductivity, and ultra-thin profiles. These properties allow engineers to create lightweight, responsive devices that bend or stretch without losing performance.
Weaknesses of Borophene Flexible Electronics
Borophene still faces challenges in large-scale synthesis, stability under ambient conditions, and device integration. Improving its resistance to air and moisture and ensuring long-term durability in real-world applications remain critical areas for future research.
Environmental Impact of Borophene Production
Borophene, a two-dimensional form of boron, shows great promise for future technologies. However, its production also raises important environmental concerns that require careful attention.
Borophene is usually produced using chemical vapor deposition (CVD) or molecular beam epitaxy (MBE). Both methods consume large amounts of energy because they require high temperatures and vacuum conditions. This energy demand increases carbon emissions, especially when manufacturers use non-renewable energy sources.
Moreover, borophene production often involves toxic precursor materials and substrates. If these chemicals are not handled properly, they could contaminate the environment. Waste materials and byproducts from borophene synthesis also need careful disposal to prevent soil and water pollution.
As the demand for borophene grows, scaling up production may create additional environmental challenges. Large-scale manufacturing can increase resource consumption and waste generation, putting pressure on natural resources.
On the positive side, borophene offers environmental benefits through its potential applications. Its high surface area and electrical conductivity make it suitable for clean energy storage and advanced water purification systems. These uses could indirectly support environmental sustainability by improving resource management and reducing pollution.
To reduce borophene’s environmental impact, researchers should explore greener production methods. Low-temperature synthesis techniques can cut energy consumption. Using renewable energy in the manufacturing process also helps minimize carbon emissions.
Additionally, scientists should focus on developing eco-friendly precursor materials. Implementing closed-loop production systems and effective recycling methods can further limit environmental harm.
Moving forward, life cycle assessments (LCA) will be crucial. These assessments help quantify the environmental footprint of borophene at every stage—from raw material extraction to waste disposal. A clear understanding of its life cycle impact can guide manufacturers toward sustainable practices.
In conclusion, while borophene offers exciting possibilities for future technology, sustainable production must be a priority. Green synthesis methods, renewable energy, and responsible waste management can ensure that borophene benefits both technology and the environment.
Borophene Commercialization Roadmap
The commercialization roadmap for borophene outlines a clear path to transform this revolutionary material from lab research into real-world applications. This journey starts with extensive fundamental research that helps scientists fully explore borophene’s unique properties and potential uses. Academic institutions and research labs play a key role in this early stage by developing synthesis methods, improving characterization techniques, and advancing theoretical models.
As research progresses, the focus shifts toward scaling up borophene production. Refining existing synthesis methods or developing new techniques is essential for large-scale manufacturing. Without scalable production, borophene cannot meet industrial demands or compete in commercial markets.
At the same time, industries must identify specific applications where borophene’s extraordinary properties offer clear advantages. Early uses will likely focus on high-value sectors like electronics, energy storage, and sensors. These fields benefit the most from borophene’s electrical conductivity, flexibility, and strength. As production becomes more cost-effective, borophene may expand into broader industries such as aerospace, automotive, and consumer electronics.
Commercialization also requires addressing several critical challenges. Companies must ensure environmental safety, compliance with regulations, and smooth integration with existing manufacturing systems. Building partnerships with other industry leaders and securing intellectual property rights will provide a competitive edge during this phase.
Strategic market entry is essential for borophene’s success. Businesses should target niche markets first to gain early traction. Once the technology matures and production costs fall, companies can move into larger and more competitive markets.
Continuous innovation is vital throughout this roadmap. Improving production techniques, enhancing borophene’s properties, and developing hybrid materials can further expand its commercial potential. Creating a robust ecosystem of suppliers, equipment manufacturers, and end-users will also help strengthen the borophene value chain.
The roadmap outlines a realistic timeline for reaching key milestones, from pilot production to mass manufacturing. It also encourages flexibility, recognizing that unexpected breakthroughs, market shifts, or regulatory updates may require adjustments along the way.
In summary, the borophene commercialization roadmap provides a strategic guide for researchers, industries, and investors. By following this clear pathway and focusing on continuous innovation, stakeholders can successfully bring borophene-based products to market and unlock the full potential of this extraordinary material.
To get detailed scientific explanations of Borophene Market Trends, try Patsnap Eureka.
