
Battery Evolution and DRS Goals
The evolution of battery technology has been a cornerstone of modern technological advancement, with lithium-ion batteries dominating the market for decades. However, as the demand for higher energy density, faster charging, and more sustainable energy storage solutions continues to grow, researchers are exploring new frontiers in battery chemistry. Disordered Rock Salts (DRS) have emerged as a promising candidate for next-generation battery materials, offering potential breakthroughs in energy storage capabilities. The primary goal of research into DRS for battery applications is to overcome the limitations of current lithium-ion technology while enhancing performance, safety, and sustainability.
Curious about breakthroughs like disordered rock salt batteries? Eureka Technical Q&A helps you explore cutting-edge materials, energy innovations, and their real-world impact—making advanced science easier to understand and apply.
DRS materials represent a paradigm shift in battery cathode design. Unlike traditional ordered crystalline structures, DRS cathodes feature a disordered arrangement of cations, which can potentially accommodate a higher concentration of working ions. This structural characteristic aims to increase energy density significantly, a critical factor in extending the range of electric vehicles and the longevity of portable electronic devices. Additionally, the disordered structure may facilitate faster ion diffusion, potentially leading to improved charging rates and overall battery performance.
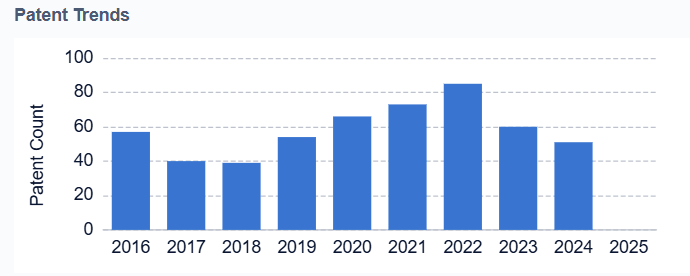
Another key objective in DRS research is to reduce reliance on cobalt, a critical component in many current lithium-ion batteries that faces supply chain challenges and ethical concerns related to mining practices. By exploring alternative compositions within the DRS framework, researchers aim to develop cobalt-free or low-cobalt cathode materials that maintain or exceed the performance of existing technologies. This aligns with broader sustainability goals in the battery industry and addresses the increasing demand for more environmentally friendly energy storage solutions.
The development of DRS materials also seeks to enhance the thermal and chemical stability of batteries, addressing safety concerns that have plagued some lithium-ion technologies. The unique structural properties of DRS may contribute to improved resistance against thermal runaway and other failure modes, potentially leading to safer battery systems for a wide range of applications, from consumer electronics to large-scale energy storage.
As research progresses, the ultimate aim is to translate the promising theoretical advantages of DRS materials into practical, commercially viable battery technologies. This involves overcoming challenges in synthesis, scaling up production, and integrating these new materials into existing battery manufacturing processes. The successful development of DRS-based batteries could revolutionize energy storage, enabling longer-lasting, faster-charging, and more sustainable power sources for the next generation of electric vehicles, renewable energy systems, and portable electronics.
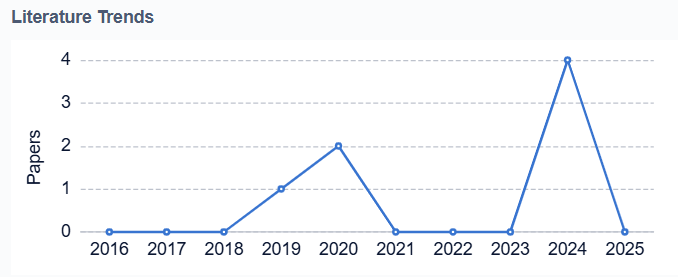
Market Demand for Advanced Batteries
The demand for advanced batteries has been growing exponentially in recent years, driven by the rapid expansion of electric vehicles (EVs), renewable energy storage systems, and portable electronic devices. This surge in demand is primarily fueled by the global push towards clean energy and sustainable transportation solutions. The EV market, in particular, has been a significant driver, with major automakers committing to electrifying their fleets in the coming decades. According to industry reports, the global EV battery market is projected to grow at a CAGR of over 25% from 2021 to 2028, reaching a value of over $175 billion by the end of the forecast period.
In addition to the automotive sector, the renewable energy industry is also contributing to the increased demand for advanced batteries. As countries worldwide aim to reduce their carbon footprint and transition to cleaner energy sources, the need for efficient and large-scale energy storage solutions has become paramount. This has led to a growing market for grid-scale battery storage systems, which is expected to reach $15 billion by 2025.
The consumer electronics sector continues to be a steady source of demand for advanced batteries, with smartphones, laptops, and wearable devices requiring increasingly powerful and long-lasting power sources. The Internet of Things (IoT) and the proliferation of smart home devices are further amplifying this demand.
Market trends indicate a strong preference for batteries with higher energy density, longer lifespan, faster charging capabilities, and improved safety features. Lithium-ion batteries currently dominate the market, but there is a growing interest in next-generation technologies that can overcome the limitations of current battery chemistries. This is where disordered rock salts and other innovative materials come into play, as they promise to deliver significant improvements in battery performance.
The industry is also witnessing a shift towards more sustainable and environmentally friendly battery technologies. Concerns over the environmental impact of battery production and disposal have led to increased research into recyclable and less toxic battery materials. This trend aligns well with the exploration of disordered rock salts, which could potentially offer a more sustainable alternative to current battery technologies.
As governments worldwide implement stricter emissions regulations and offer incentives for clean energy adoption, the demand for advanced batteries is expected to continue its upward trajectory. This creates a fertile ground for breakthrough technologies like disordered rock salts to enter the market and potentially revolutionize the energy storage landscape.
DRS Challenges and Limitations
Disordered rock salts (DRS) have emerged as a promising class of materials for next-generation battery cathodes, offering potential breakthroughs in energy density and performance. The current state of DRS research is characterized by rapid advancements and significant challenges. Globally, research efforts are concentrated in leading academic institutions and industrial R&D centers, with notable progress in countries like the United States, China, Japan, and South Korea.
The primary attraction of DRS lies in their ability to potentially double the energy density of lithium-ion batteries. This is achieved through their unique crystal structure, which allows for a higher concentration of transition metal ions compared to conventional cathode materials. However, the development of DRS faces several critical challenges. One major obstacle is the inherent structural instability during cycling, leading to capacity fade and reduced battery lifespan. Researchers are actively working on strategies to mitigate this issue, including doping with stabilizing elements and surface modifications.
Another significant challenge is the relatively low ionic and electronic conductivity of DRS materials. This limitation impacts the rate capability and overall performance of batteries incorporating these cathodes. To address this, scientists are exploring various approaches, such as nanostructuring, conductive coatings, and the introduction of artificial conduction pathways.
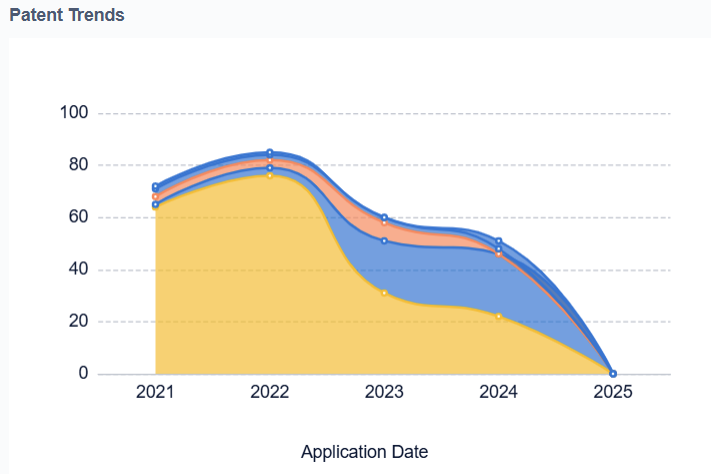
The synthesis of high-quality DRS materials at scale remains a technical hurdle. Current methods often result in inhomogeneous compositions or unwanted impurities, which can negatively affect battery performance. Developing scalable and cost-effective synthesis techniques that maintain material quality is crucial for the commercial viability of DRS-based batteries.
Environmental concerns and resource availability also pose challenges to the widespread adoption of DRS technology. Many DRS compositions rely on critical elements like cobalt and nickel, which face supply chain issues and sustainability concerns. Consequently, there is a growing focus on developing DRS materials with reduced or eliminated dependence on these elements.
Despite these challenges, the field is witnessing rapid progress. Recent breakthroughs include the development of cation-disordered oxyfluorides with improved stability and the discovery of novel compositions with enhanced electrochemical properties. Computational methods and advanced characterization techniques are playing increasingly important roles in accelerating the discovery and optimization of DRS materials.
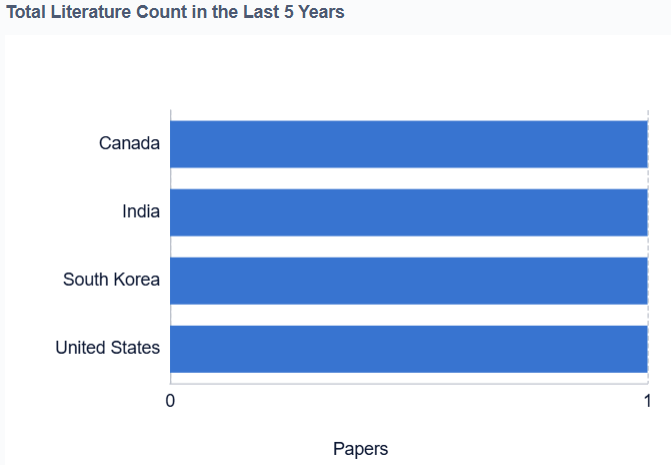
The geographical distribution of DRS research shows concentrations in regions with strong battery industry presence and government support for energy storage technologies. While academic institutions lead in fundamental research, collaborations with industry partners are becoming more common, aiming to bridge the gap between laboratory discoveries and commercial applications.
Battery Technology Roadmap
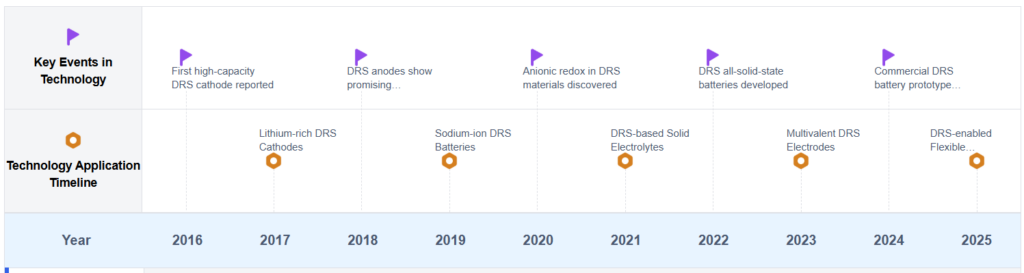
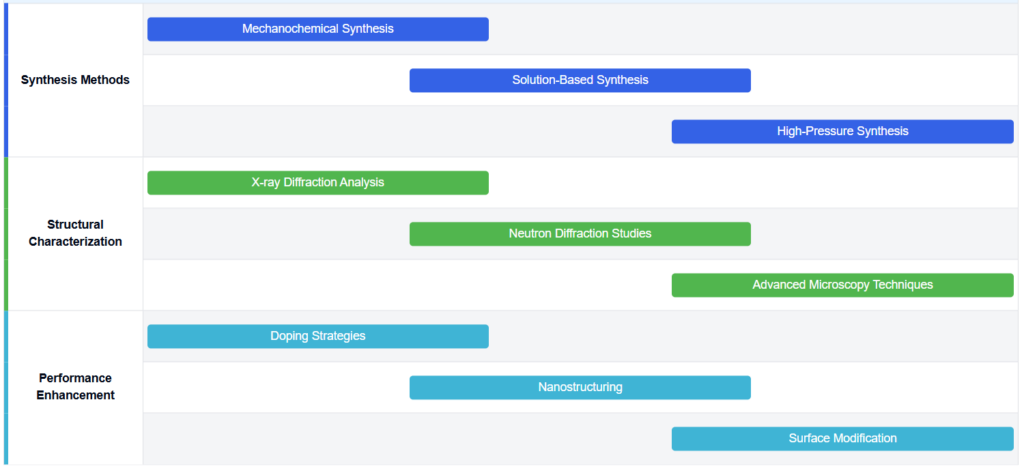
Key Players in DRS Research
The research on disordered rock salts for battery breakthroughs is in an emerging stage, with significant potential for market growth. The technology is still in its early phases of development, with a mix of academic institutions and industry players driving innovation. Companies like Wildcat Discovery Technologies, Dyson Technology, and LG Chem are actively involved in advancing this technology. The market size is expected to expand rapidly as the demand for high-performance batteries increases across various sectors. While the technology shows promise, it is not yet fully mature, with ongoing research at institutions such as the University of California, Massachusetts Institute of Technology, and Central South University focusing on improving performance and scalability.
Wildcat Discovery Technologies, Inc.
Technical Solution
Wildcat Discovery Technologies has developed a high-throughput materials discovery platform specifically tailored for disordered rock salt materials. Their approach combines advanced computational modeling with rapid experimental synthesis and characterization techniques. This allows them to explore a vast compositional space of disordered rock salts, identifying promising candidates with enhanced ionic conductivity and structural stability. Their platform can rapidly iterate through thousands of compositions, optimizing for factors such as lithium diffusion pathways, voltage stability, and cycling performance.
Strengths: Rapid exploration of vast material space, data-driven optimization. Weaknesses: May overlook unconventional compositions, high initial investment in technology.
The Regents of the University of California
Technical Solution
The University of California system has been at the forefront of disordered rock salt research for battery applications. Their approach focuses on understanding the fundamental mechanisms of ion transport in these materials. They have developed advanced characterization techniques, including in-situ X-ray diffraction and neutron scattering, to probe the local structure and dynamics of disordered rock salts during battery operation. Additionally, they have pioneered the use of first-principles calculations to predict and design new disordered rock salt compositions with improved electrochemical properties.
Strengths: Deep fundamental understanding, cutting-edge characterization techniques. Weaknesses: Potential gap between academic research and industrial application.
Massachusetts Institute of Technology
Technical Solution
MIT’s research on disordered rock salts for battery breakthroughs focuses on developing novel synthesis methods and understanding structure-property relationships. They have pioneered the use of mechanochemical synthesis to create disordered rock salts with precise control over composition and disorder. Their approach also includes advanced computational modeling to predict the electrochemical properties of these materials. MIT researchers have developed a unique “cation-disordered” oxides framework, which allows for higher energy density and improved rate capability compared to traditional cathode materials.
Strengths: Innovative synthesis methods, strong computational modeling capabilities. Weaknesses: May face challenges in scaling up laboratory processes for industrial production.
LG Chem Ltd.
Technical Solution
LG Chem has been actively researching disordered rock salts as part of their next-generation battery material development program. Their approach combines high-throughput experimental screening with advanced characterization techniques. They have developed proprietary synthesis methods to create disordered rock salts with optimized compositions for high energy density and long cycle life. LG Chem’s research also focuses on integrating these materials into full cell designs, addressing challenges such as electrolyte compatibility and electrode engineering.
Strengths: Strong integration with existing battery manufacturing capabilities, focus on practical application. Weaknesses: May be constrained by current battery design paradigms.
Current DRS Battery Solutions
Composition of disordered rock salt cathode materialsDisordered rock salt cathode materials are being developed to improve battery performance. These materials typically consist of lithium, transition metals, and oxygen in a disordered structure. The composition can be optimized to enhance capacity, cycling stability, and rate capability of batteries.
- Composition of disordered rock salt cathode materials
Researchers design disordered rock salt cathode materials using various elements, including transition metals, lithium, and oxygen. They tailor the composition by adjusting the ratios of elements like nickel, manganese, cobalt, and other dopants to optimize battery performance. The disordered structure allows for higher lithium content and improved ionic conductivity compared to traditional layered cathode materials. - Synthesis methods for disordered rock salt cathodes
Various synthesis methods are employed to produce disordered rock salt cathode materials, including solid-state reactions, sol-gel processes, and mechanochemical synthesis. These methods aim to create a homogeneous distribution of elements and maintain the disordered structure. Controlling synthesis parameters such as temperature, pressure, and precursor selection is crucial for achieving desired material properties and battery performance. - Doping strategies to enhance battery performance
Doping disordered rock salt cathode materials with various elements can significantly improve battery performance. Common dopants include fluorine, chlorine, sulfur, and transition metals. These dopants can enhance structural stability, increase capacity, improve rate capability, and extend cycle life. The selection and concentration of dopants are optimized based on their effects on the material’s electronic structure and lithium diffusion pathways. - Surface modification and coating techniques
Surface modification and coating of disordered rock salt cathode particles can enhance their electrochemical performance and stability. Techniques such as atomic layer deposition, solution-based coating, and in-situ surface treatment are used to create protective layers or modify the surface chemistry. These modifications can reduce side reactions with the electrolyte, improve interfacial stability, and enhance the overall battery performance.
Doping strategies for disordered rock salt cathodes
Doping disordered rock salt cathodes with various elements can significantly improve their electrochemical properties. Common dopants include fluorine, sulfur, and other transition metals. These dopants can enhance ionic conductivity, structural stability, and overall battery performance.Expand
Synthesis methods for disordered rock salt materials
Various synthesis methods are employed to produce high-performance disordered rock salt materials. These include sol-gel methods, solid-state reactions, and mechanochemical processes. The choice of synthesis method can greatly influence the material’s properties and battery performance.Expand
Surface modification of disordered rock salt cathodes
Surface modification techniques are applied to disordered rock salt cathodes to improve their stability and performance. These modifications can include coatings, surface treatments, or the creation of core-shell structures. Such modifications can enhance the cathode-electrolyte interface stability and improve cycling performance.Expand
Integration of disordered rock salts in battery systems
The integration of disordered rock salt cathodes into full battery systems is being explored to maximize their potential. This includes optimizing electrolyte compositions, anode materials, and cell designs to complement the unique properties of disordered rock salt cathodes and enhance overall battery performance.
Core DRS Innovations
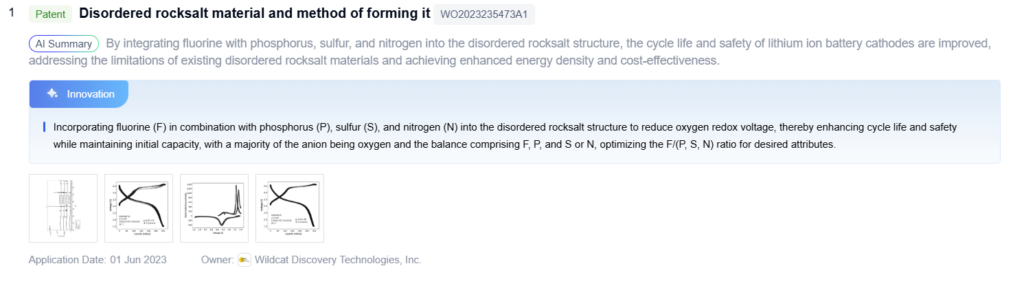
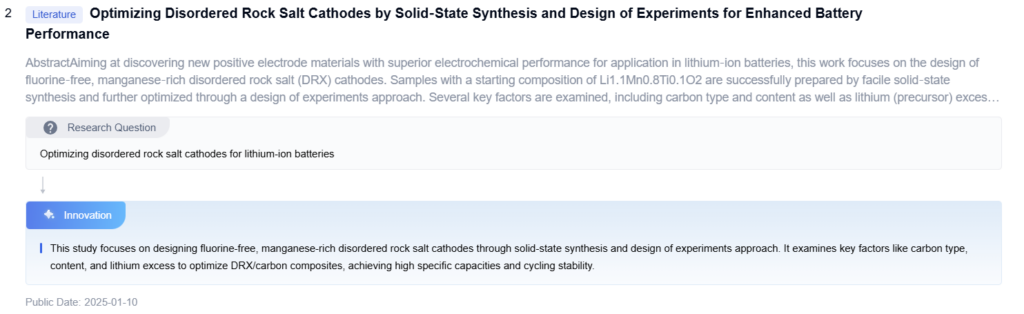
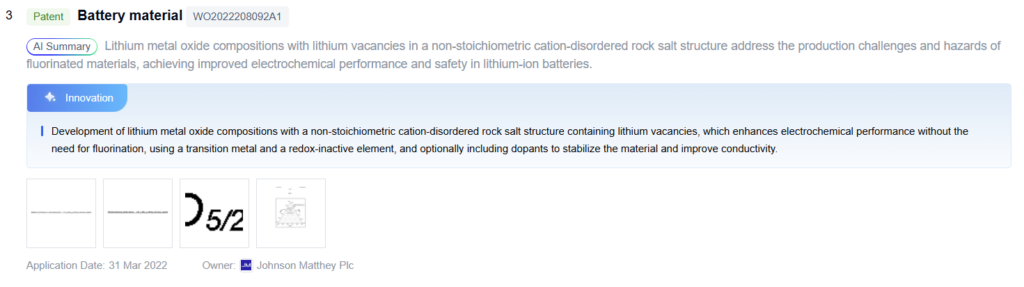
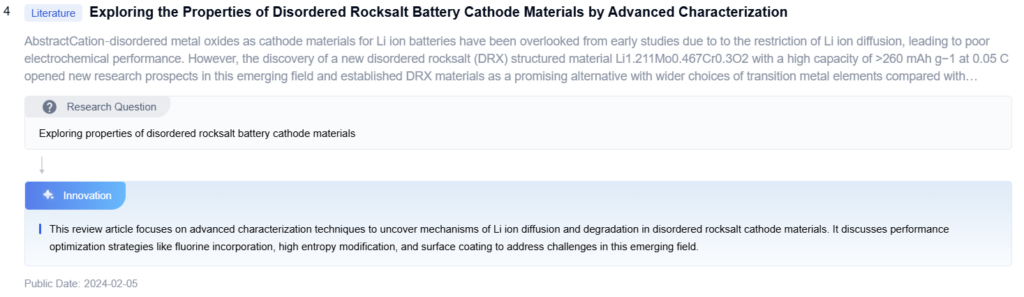
Future DRS Research Directions
Advanced Synthesis Techniques for Disordered Rock Salts
Advanced synthesis methods for disordered rock salts (DRS) are unlocking new potential in battery research. These techniques aim to improve electrochemical performance, structural stability, and scalability of DRS cathode materials.
Mechanochemical Synthesis
Researchers use high-energy ball milling to induce disorder in precursor materials. This process forms metastable phases and allows for the incorporation of diverse elements into the rock salt structure. It offers a powerful way to fine-tune material composition and electrochemical properties.
Solution-Based Methods
Scientists apply co-precipitation and sol-gel techniques, followed by heat treatment, to control particle size and morphology. These methods deliver better composition uniformity and improve performance consistency in DRS materials.
Spray Pyrolysis Techniques
Spray pyrolysis and flame spray pyrolysis offer scalable routes to synthesize DRS with nanoscale structures. These methods enable high throughput production while enhancing electrochemical behavior.
In-Situ Synthesis in Batteries
Researchers are exploring in-situ synthesis, which forms DRS structures during the battery’s first charge-discharge cycle. This approach may simplify manufacturing and improve the electrode-electrolyte interface.
Doping Strategies
Doping DRS materials with elements like fluorine, magnesium, or zirconium helps stabilize the structure. It also enhances lithium-ion diffusion and reduces cation disorder, boosting overall battery performance.
Composite Material Integration
Combining DRS with conductive materials like graphene or carbon nanotubes addresses electronic conductivity limitations. These composites maintain high capacity while improving cycle stability and rate performance.
Strengths
- Enables design of cathode materials with customizable properties
- Supports high energy density
- Offers precise control over structure and composition
Weaknesses
- Some methods face scalability challenges
- Specialized equipment can drive up costs
- Reproducing specific disorder levels at scale remains complex
Environmental Impact of DRS
Disordered Rock Salts (DRS) show strong potential in next-generation batteries. However, their environmental impact must be carefully managed to ensure a sustainable energy transition.
Resource Efficiency and Material Sourcing
DRS materials allow the use of a broader range of elements. This flexibility can reduce reliance on rare earth metals and other scarce resources. By avoiding critical materials, DRS production may lower the environmental damage caused by mining.
Energy Use in Manufacturing
The complex structure of DRS materials can require energy-intensive synthesis processes. High-temperature reactions or extended milling steps may increase energy demand, which could offset some sustainability gains. Life cycle assessments are essential to evaluate the true environmental cost of DRS batteries compared to traditional lithium-ion batteries.
Longer Battery Life and Reduced Waste
One of the biggest advantages of DRS batteries is improved energy density and cycle life. This means users may replace batteries less frequently, cutting down on material consumption and long-term waste. Fewer replacements also reduce emissions tied to manufacturing and transportation.
Enabling Renewable Energy Storage
DRS batteries could help stabilize power grids by enabling large-scale energy storage. With better performance and durability, they can store more renewable energy, reducing reliance on fossil fuels. This benefit contributes to long-term emissions reductions across multiple sectors.
Recycling and End-of-Life Considerations
The complex chemistry of DRS poses recycling challenges. Traditional recycling methods may not work well for these materials. However, their unique composition also creates opportunities for innovation in recovery technologies. Researchers are developing processes to reclaim valuable elements and minimize environmental harm.
Potential Risks and Safety Management
As researchers introduce new elements into DRS compositions, they must monitor for environmental or health risks. Manufacturers must implement safe handling, disposal protocols, and material safety reviews to prevent unintended consequences.
Moving Toward Sustainable DRS Batteries
To ensure DRS development stays sustainable, manufacturers must:
- Optimize energy use in production
- Improve recyclability and reduce waste
- Source materials responsibly
- Monitor environmental impacts continuously
Safety Considerations for DRS Batteries
Safety remains a top priority in the development and deployment of disordered rock salt (DRS) batteries. As this new technology nears commercialization, researchers and manufacturers must carefully manage potential risks and implement solid safety measures. One major concern is thermal stability. DRS materials must remain stable under high temperatures and stressful conditions. To ensure this, researchers conduct thermal runaway tests that examine how DRS batteries behave across different temperature ranges and during extreme use.
Chemical interactions between DRS materials and electrolytes also demand close attention. Unwanted reactions could trigger gas formation or accelerate capacity loss, both of which compromise safety and performance. Mechanical integrity is another area of focus. Ion insertion and extraction during charging and discharging can cause the electrodes to expand and contract. Over time, this can lead to cracks, delamination, or even internal short circuits. To reduce these risks, engineers explore advanced electrode designs and protective coatings that stabilize the structure through repeated cycles.
Dendrite formation poses a further hazard. If needle-like structures grow inside the battery, they could pierce separators and cause dangerous short circuits. Researchers must investigate this risk thoroughly and develop solutions if needed. The manufacturing process for DRS batteries must also maintain strict quality control. Impurities or microscopic flaws introduced during synthesis or assembly can affect both safety and performance. Standardized procedures and quality assurance systems are essential for delivering reliable, safe batteries at scale.
Environmental and health factors also deserve careful consideration. Developers must evaluate the toxicity of DRS materials and their byproducts to ensure safe handling throughout the battery’s life cycle—from production to disposal. They must also assess the risk of hazardous leaching and plan responsible recycling strategies to minimize environmental harm.
As DRS batteries enter commercial markets, specific safety testing protocols will be needed. These should include abuse tests for scenarios like overcharging, deep discharging, and physical damage. Long-term cycle testing under varying temperatures and humidity levels will also help gauge how well DRS batteries hold up over time. Collaboration will play a key role in this process. Industry leaders, researchers, and regulators must work together to adapt existing safety regulations and possibly create new standards tailored to DRS technology.
By making safety an integral part of the development process, stakeholders can ensure that DRS batteries offer high performance without compromising user protection or environmental responsibility.
To get detailed scientific explanations of disordered rock salts, try Patsnap Eureka.
