
Graphene Oxide Evolution and Research Objectives
Graphene oxide, a graphene derivative, has emerged as a breakthrough material with wide-ranging potential across many industries. Researchers discovered its promise in the early 2000s and have since driven rapid development in synthesis and applications. Interest continues to grow due to its unique structure, chemical tunability, and functional versatility.
Exploring the future of graphene oxide? Eureka Technical Q&A offers expert insights into its properties, applications, and emerging trends—helping you unlock its potential in energy storage, electronics, biomedicine, and beyond.
Early efforts focused on refining production methods like the modified Hummers method, which remains widely used for bulk synthesis. As scientists improved these methods, they began exploring graphene oxide’s electrical, mechanical, and thermal behavior in greater detail. These studies revealed how structural features influence its performance, guiding efforts to tailor its properties for specific uses.
Over time, research goals evolved to meet market demands and improve understanding of the material. Initial work centered on optimizing synthesis and characterizing key physical and chemical traits. As new applications emerged, researchers shifted toward developing functional graphene oxide materials and integrated devices.
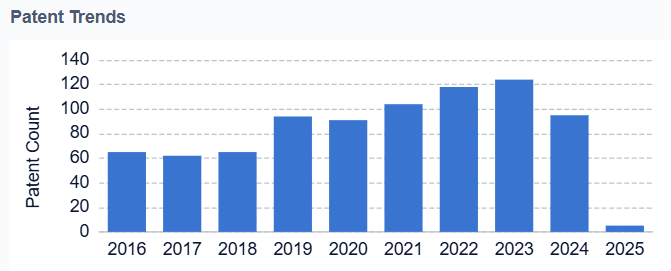
Today’s research targets large-scale production, precise oxygen control, and seamless integration into industrial manufacturing systems. Scientists also explore innovative uses in energy storage, water treatment, biomedicine, and flexible electronics. These applications highlight graphene oxide’s broad impact on both emerging and traditional technologies.
Looking ahead, future research will likely focus on performance enhancements, environmental sustainability, and hybrid materials. Scientists aim to combine graphene oxide with other nanomaterials to create new multifunctional composites. Interdisciplinary collaboration among chemists, engineers, and physicists will play a key role in realizing commercial success.
As interest expands, graphene oxide will continue driving innovation across diverse sectors, from clean energy to healthcare and next-generation electronics.
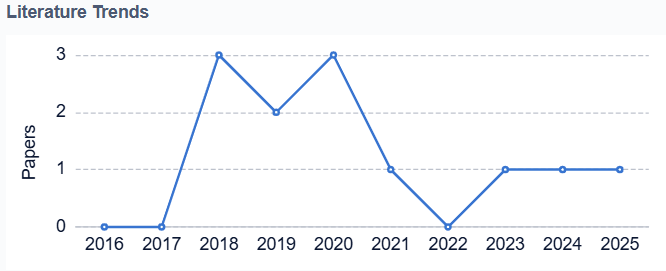
Market Demand Analysis for Graphene Oxide
The graphene oxide market has grown rapidly in recent years and continues to expand across multiple industries. This growth stems from its unique properties and wide-ranging applications.
In electronics, manufacturers use graphene oxide to develop flexible displays, touchscreens, and next-generation batteries. The automotive industry explores it to improve electric vehicle battery performance and create lighter composite materials.
In healthcare, researchers apply graphene oxide in drug delivery, biosensors, and tissue engineering. Environmental sectors use it for water purification and air filtration technologies. In construction, companies study its use in concrete reinforcement and anti-corrosion coatings.
In 2020, the global graphene oxide market reached around USD 220 million. Analysts expect it to grow to USD 1.3 billion by 2028. This reflects a strong CAGR of approximately 22% during the forecast period.
Asia-Pacific, led by China and South Korea, dominates this market. Government support and heavy R&D investments fuel this regional growth. North America and Europe also play major roles, thanks to key industry players and growing demand in healthcare and electronics.
Rising interest in sustainability also drives market expansion. Graphene oxide offers eco-friendly solutions for energy storage and environmental cleanup.
However, some challenges remain. High production costs and lack of standardization slow adoption. Scaling up manufacturing also presents technical hurdles.
Despite these issues, market prospects remain strong. Ongoing research aims to lower costs, improve scalability, and unlock more applications. The future of graphene oxide looks bright across industries like energy, electronics, and healthcare.
Current Challenges in Graphene Oxide Production
Despite its impressive potential, graphene oxide (GO) still faces major challenges in large-scale production and commercialization. One key issue is the lack of standardized production methods, which leads to inconsistent quality across batches. These inconsistencies hinder research reproducibility and complicate industrial use.
Current production techniques also require high energy input and harsh chemicals, generating hazardous waste. These factors drive up costs and reduce GO’s competitiveness. Scaling up from lab to industrial production adds further complexity, especially when producing large, defect-free GO sheets.
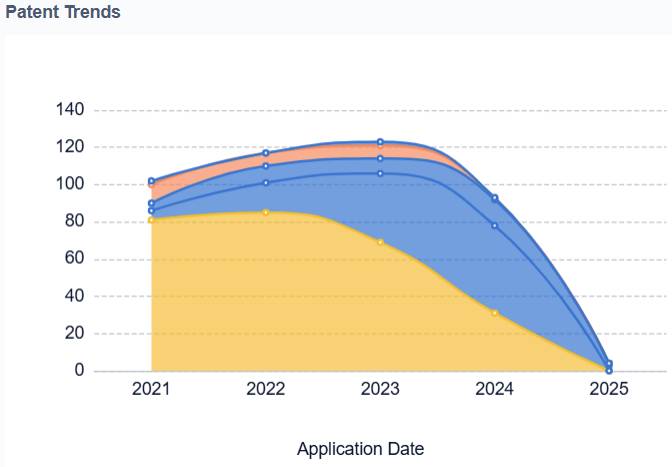
Maintaining control over oxygen content and surface functional groups is another major hurdle. These factors directly affect GO’s performance, yet remain difficult to regulate at scale.
GO also suffers from poor stability. It tends to agglomerate or reduce over time, which alters its properties and shortens shelf life. This instability poses challenges for storage, shipping, and integration into end products.
Health and safety concerns further complicate GO adoption. Researchers still don’t fully understand the long-term effects of nanoparticle exposure. These unknowns have triggered regulatory hurdles in several regions.
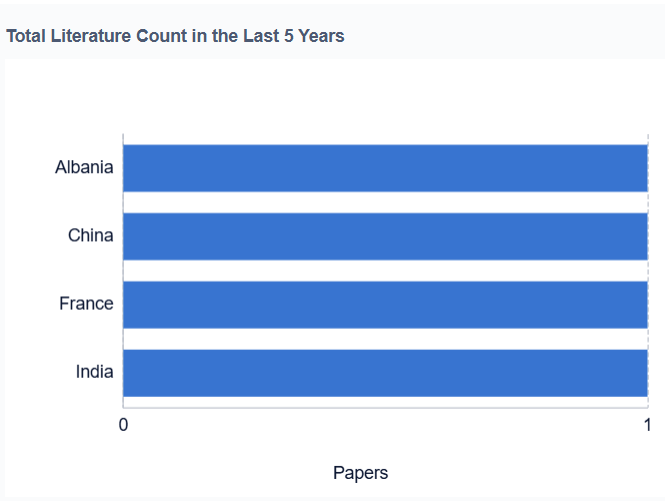
Additionally, developing cost-effective methods to reduce GO to graphene remains difficult. Precision control over the reduction process is essential to tailor GO’s properties for specific uses.
Overcoming these technical, environmental, and regulatory challenges is critical. Doing so will unlock GO’s full potential and drive broader commercial adoption across industries.
Graphene Oxide Development Timeline
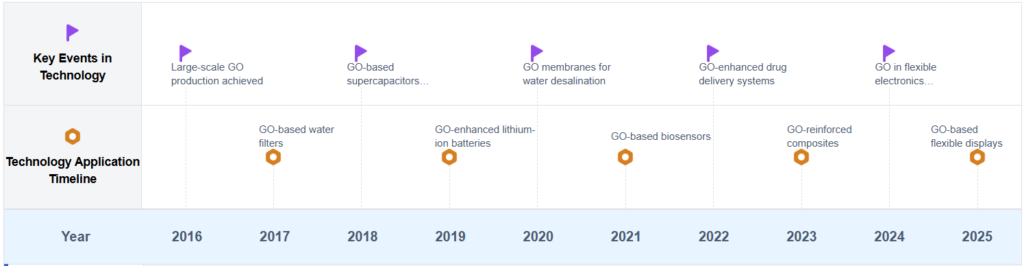
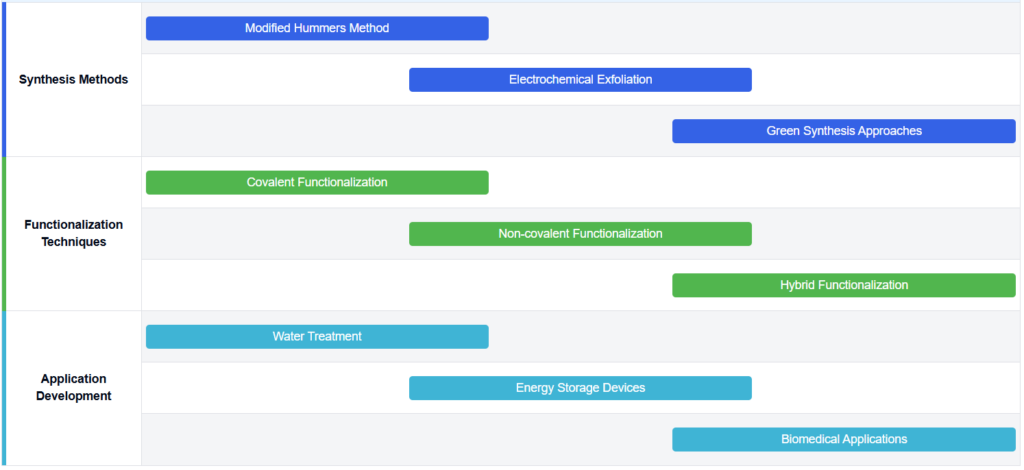
Key Players in Graphene Oxide Industry
The graphene oxide market is in a growth phase, driven by increasing applications across various industries. The market size is expanding rapidly, with projections indicating significant growth in the coming years. Technologically, graphene oxide is advancing from early-stage development to more mature applications. Key players like China Petroleum & Chemical Corp., Nanotek Instruments, and Zhejiang University are leading research efforts, while institutions such as William Marsh Rice University and Centre National de la Recherche Scientifique contribute to scientific advancements. Companies like Toray Industries and Nitto Denko Corp. are focusing on industrial applications, indicating a shift towards commercialization. The competitive landscape is diverse, with a mix of academic institutions, research centers, and industrial players, suggesting a collaborative yet competitive environment for innovation and market development.
China Petroleum & Chemical Corp.
Technical Solution
China Petroleum & Chemical Corp. (Sinopec) has developed a proprietary graphene oxide production method using a modified Hummers’ process. Their technique involves careful control of oxidation conditions and exfoliation parameters to produce high-quality graphene oxide sheets with controlled thickness and lateral dimensions. Sinopec has also implemented a scalable production line capable of producing tons of graphene oxide annually, making it suitable for various industrial applications.
Strengths: Large-scale production capability, consistent quality control, and integration with existing petrochemical infrastructure.
Weaknesses: Potential environmental concerns related to chemical processes and energy consumption.
Nanotek Instruments, Inc.
Technical Solution
Nanotek Instruments has developed a novel approach to graphene oxide production using a combination of electrochemical exfoliation and controlled oxidation. Their process allows for the creation of graphene oxide with tailored oxygen content and functional groups. The company has also pioneered the development of graphene oxide-based composites for energy storage applications, particularly in supercapacitors and lithium-ion batteries. Their technology enables the production of graphene oxide with high surface area and excellent electrical conductivity.
Strengths: Customizable graphene oxide properties, focus on high-value applications in energy storage. Weaknesses: Limited large-scale production capabilities compared to larger corporations.
Sichuan Institute of Graphene Industrial Technology
Technical Solution
The Sichuan Institute has developed an environmentally friendly graphene oxide production method using natural graphite as a raw material. Their process involves a combination of mechanical exfoliation and mild chemical oxidation, reducing the use of harsh chemicals. The institute has also created a range of graphene oxide-based products, including conductive inks, coatings, and polymer composites. Their research focuses on improving the dispersion and compatibility of graphene oxide in various matrices for enhanced material properties.
Strengths: Eco-friendly production process, diverse range of graphene oxide applications.
Weaknesses: Potential limitations in achieving high oxidation levels compared to traditional methods.
Toray Industries, Inc.
Technical Solution
Toray Industries has developed a proprietary graphene oxide production method that combines liquid-phase exfoliation with controlled oxidation. Their process allows for the creation of graphene oxide with precise control over flake size and oxidation degree. Toray has also invested in the development of graphene oxide-based membranes for water purification and gas separation applications. Their technology enables the production of large-area graphene oxide films with controlled porosity and surface functionality.
Strengths: Advanced membrane technology applications, integration with existing materials science expertise.
Weaknesses: Potential high production costs due to precision control requirements.
The University of Manchester
Technical Solution
The University of Manchester, birthplace of graphene, has developed several innovative approaches to graphene oxide production and application. Their research includes the use of electrochemical exfoliation techniques to produce graphene oxide with controlled defect density and oxygen content. The university has also pioneered the development of graphene oxide-based sensors and biomedical applications, including drug delivery systems and tissue engineering scaffolds. Their work focuses on understanding the fundamental properties of graphene oxide and exploiting its unique characteristics for advanced applications.
Strengths: Cutting-edge research in graphene oxide properties and applications, strong academic collaborations.
Weaknesses: Potential challenges in scaling up laboratory techniques to industrial production.
Current Graphene Oxide Applications
Production and synthesis methods
Various techniques for producing and synthesizing graphene oxide are being developed and improved. These methods aim to enhance the quality, yield, and scalability of graphene oxide production, which is crucial for meeting the growing market demand. Innovations in this area focus on optimizing exfoliation processes, chemical treatments, and purification techniques.
- Market analysis and trend forecasting for graphene oxide
Various patents focus on analyzing market trends and forecasting future developments in the graphene oxide industry. These methods involve data collection, processing, and analysis to predict market behavior, consumer preferences, and potential growth areas. Such analyses help businesses make informed decisions regarding production and investment in graphene oxide technologies. - Production and synthesis methods for graphene oxide
Several patents describe innovative methods for producing and synthesizing GO. These techniques aim to improve the efficiency, quality, and scalability of GO production. The methods may involve chemical, mechanical, or electrochemical processes, as well as novel approaches to exfoliation and oxidation of graphite precursors. - Applications and market opportunities for GO
Patents in this category explore various applications and potential market opportunities for graphene oxide. These may include its use in electronics, energy storage, water purification, biomedical applications, and advanced materials. The patents discuss how these applications can drive market growth and create new business opportunities in the GO industry.
Applications in energy storage
GO is increasingly being utilized in energy storage devices, particularly in batteries and supercapacitors. Its unique properties, such as high surface area and excellent conductivity, make it an attractive material for improving the performance and efficiency of these devices. Research is ongoing to develop new graphene oxide-based composites and structures for enhanced energy storage capabilities.
Environmental and water treatment applications
The market for GO in environmental applications, especially water treatment, is expanding. Its adsorption properties make it effective for removing contaminants from water. Ongoing research focuses on developing graphene oxide-based membranes and filters for water purification, desalination, and waste treatment processes.
Biomedical and healthcare applications
GO is finding increasing applications in the biomedical and healthcare sectors. Its unique properties are being exploited for drug delivery systems, biosensors, tissue engineering, and antimicrobial coatings. Research trends indicate growing interest in developing graphene oxide-based materials for targeted cancer therapies and advanced diagnostic tools.
Composite materials and coatings
The use of GO in composite materials and coatings is a significant market trend. It is being incorporated into polymers, ceramics, and metals to enhance their mechanical, thermal, and electrical properties. Research is focused on developing new graphene oxide-based composites for applications in aerospace, automotive, and construction industries.
Breakthrough Technologies in Graphene Oxide Synthesis
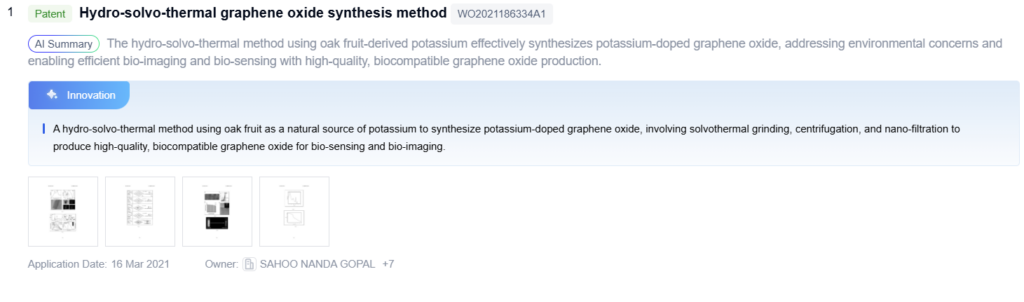
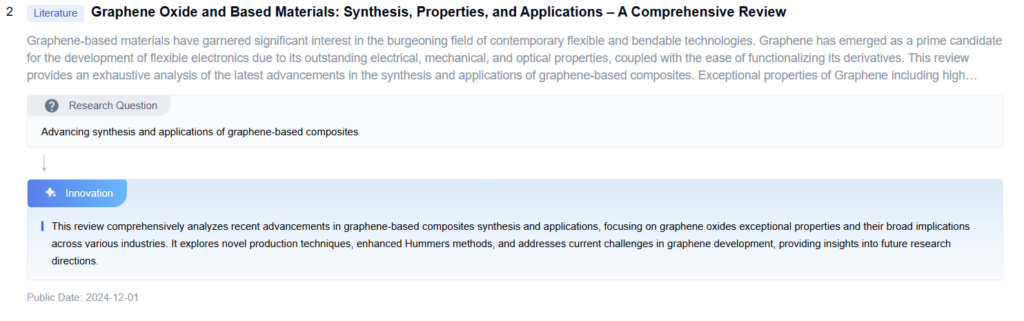
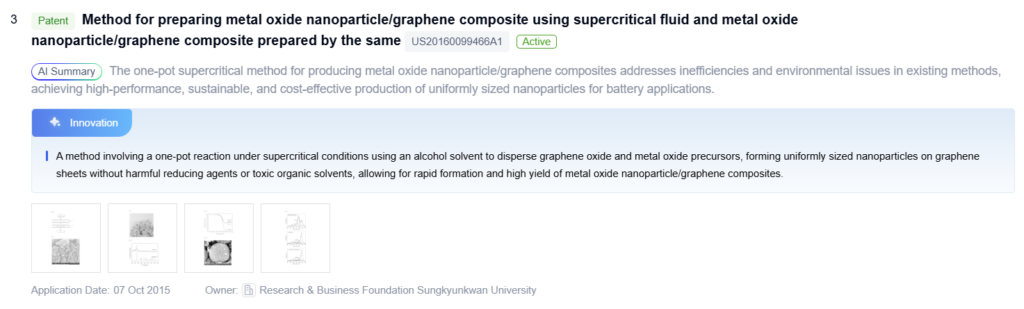
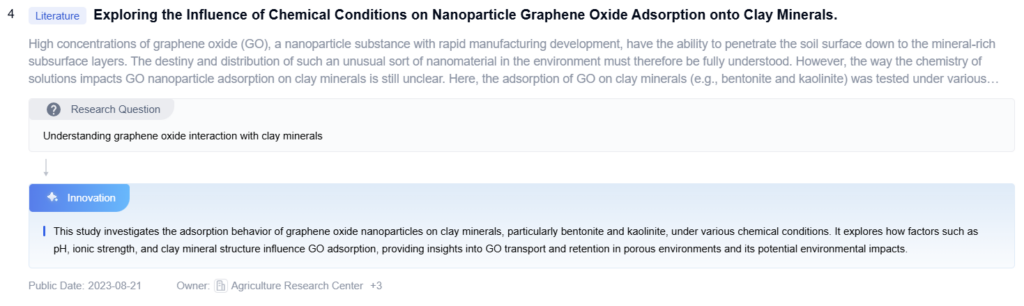
Future Innovations in Graphene Oxide
Advanced Graphene Oxide-based Energy Storage Systems
Graphene oxide (GO) is driving the next generation of energy storage solutions with enhanced performance and flexibility. Its unique properties—large surface area, excellent conductivity, and high mechanical strength—make it ideal for high-performance batteries and supercapacitors.
Researchers use GO to design advanced electrodes with optimized porosity and surface chemistry. These engineered structures allow faster ion transport and higher energy density. By combining GO with materials like metal oxides or conductive polymers, they create composites with improved power output and cycle life.
A major innovation involves flexible and stretchable GO-based storage devices. These units integrate easily into wearables, smart textiles, and foldable electronics. GO’s flexibility enables thin, lightweight energy units that bend and twist without performance loss.
GO also enhances solid-state battery designs. Scientists incorporate it into polymer or ceramic electrolytes to improve stability and safety. These solid-state batteries reduce the risk of leakage and fire, while boosting energy density and longevity.
To enable widespread adoption, manufacturers are improving the scalability of GO production. They focus on greener synthesis methods and more efficient manufacturing processes to cut costs and environmental impact.
Strengths:
- Delivers higher energy and power densities than traditional systems
- Supports faster charging and longer cycle life
- Enables flexible, lightweight, and wearable devices
- Enhances safety in next-generation solid-state batteries
Weaknesses:
- Producing high-quality GO remains expensive
- Scaling up manufacturing poses technical challenges
- Environmental impact from GO production and disposal needs further assessment
Graphene Oxide-based Environmental Remediation Technologies
Graphene oxide (GO) is transforming environmental remediation, especially in water and air purification systems. Its large surface area, tunable chemistry, and high adsorption capacity make it a standout material in green technology.
In water treatment, GO membranes offer advanced filtration capabilities. These membranes remove heavy metals, organic pollutants, and even microorganisms with high precision. Thanks to their excellent permeability and selectivity, GO membranes enable fast and efficient purification. They show promise in both desalination and industrial wastewater treatment.
GO also excels as an adsorbent. Researchers modify it with functional groups or nanoparticles to capture specific pollutants. This approach targets contaminants like dyes, pesticides, and toxic metals in groundwater and industrial effluents. The customizability of GO adsorbents makes them ideal for selective and efficient pollutant removal.
In air purification, GO-based materials play a crucial role. Integrated into filters or surface coatings, they trap volatile organic compounds (VOCs), dust, and other pollutants. Some GO composites also use light to break down toxins, making them suitable for self-cleaning surfaces.
Environmental sensors based on GO are gaining attention too. These devices detect trace pollutants in water or air with high sensitivity. Their compact size and responsiveness make them perfect for smart city infrastructure and real-time environmental monitoring.
GO even shows potential in soil remediation. When added to soil, it can immobilize contaminants and improve water retention. This dual function supports both environmental recovery and sustainable agriculture.
Strengths:
- Removes a wide range of water and air pollutants
- Supports targeted remediation through functionalization
- Enables dual-use applications like sensing and filtering
- Offers potential for reusable, self-cleaning technologies
Weaknesses:
- High production costs slow down mass adoption
- Risk of nanoparticle leakage into ecosystems
- Recovery and recycling of GO remains challenging
- Regulatory uncertainties hinder large-scale deployment
Environmental Impact of Graphene Oxide
Graphene oxide (GO) is gaining traction for its role in environmental cleanup, but its growing use raises important ecological questions. As industries embrace GO for its high surface area and powerful adsorption capabilities, understanding its full environmental footprint becomes essential.
GO shows great promise in water purification. It can effectively remove heavy metals, organic pollutants, and other harmful substances from water. GO-based membranes have demonstrated excellent performance, offering potential breakthroughs in desalination and wastewater treatment. These innovations could significantly improve access to clean water worldwide.
However, GO’s benefits come with potential risks. When released into the environment—whether during manufacturing or application—GO particles may interact with organisms and ecosystems in harmful ways. Studies show GO can accumulate in aquatic life, possibly causing oxidative stress or other biological damage. Long-term effects on both aquatic and terrestrial systems remain unclear, highlighting the need for more research.
The environmental cost of GO production also deserves attention. The widely used Hummers method relies on harsh chemicals that create hazardous waste. To address this, scientists are exploring greener synthesis techniques like electrochemical exfoliation and sustainable reduction processes.
Another concern is GO’s ability to carry adsorbed pollutants through soil and water systems. While this property makes GO useful in remediation, it might also help contaminants spread, increasing their environmental impact and toxicity.
As GO enters more commercial products, end-of-life considerations become urgent. GO may persist in ecosystems and bioaccumulate over time. Without proper recycling and disposal practices, this could pose future environmental challenges.
Graphene Oxide Standardization and Quality Control
Graphene oxide (GO) standardization is becoming increasingly critical as industries push for broader commercial use. Consistent quality and reliable specifications are essential for scaling GO-based technologies across electronics, biomedicine, and environmental applications. Yet, the GO market still lacks universally accepted standards for production, characterization, and quality assurance.
To bridge this gap, international organizations and leading research institutions are actively working to establish standardized protocols. Their goal is to create a common framework that ensures reproducibility, enables product comparisons, and simplifies regulatory approval. Key properties under review include lateral size distribution, thickness, oxygen content, and defect density.
One major challenge lies in the variability of GO synthesis. Different production techniques—like the modified Hummers method or electrochemical exfoliation—can yield GO with vastly different characteristics. Without standardized procedures, manufacturers face difficulties maintaining consistency, while users struggle to compare products reliably.
To address this, researchers are developing detailed production protocols that promote uniformity across batches and suppliers. They’re also implementing in-line quality control tools, such as real-time spectroscopic monitoring, to assess oxidation and exfoliation levels during synthesis.
Post-production analysis is equally vital. Techniques like Raman spectroscopy, X-ray photoelectron spectroscopy (XPS), and atomic force microscopy (AFM) are being standardized to deliver consistent data on GO structure and composition. These tools help validate quality and build trust in product performance.
The creation of certified reference materials plays a key role as well. National metrology institutes and standards bodies are collaborating to develop GO samples with well-defined properties. These serve as calibration tools for equipment and benchmarks for quality testing.
As the field advances, international standards organizations such as ISO and ASTM are expected to formalize GO guidelines. These standards will likely define terminology, measurement methods, and performance criteria. Adoption of such standards will streamline industry practices, improve product quality, and enhance market credibility.
To get detailed scientific explanations of graphene oxide, try Patsnap Eureka.
