
What is the Otto Cycle?
The Otto cycle is a cornerstone of thermodynamics, explaining how spark-ignition internal combustion engines work. Developed by in the late 19th century, this cycle underpins modern gasoline engine design. It features four key processes: two adiabatic (isentropic) and two isochoric (constant volume) stages. This article will explore the principles, processes, efficiency, and real-world applications of the Otto cycle, providing a comprehensive understanding of its role in engine technology and thermodynamic analysis.
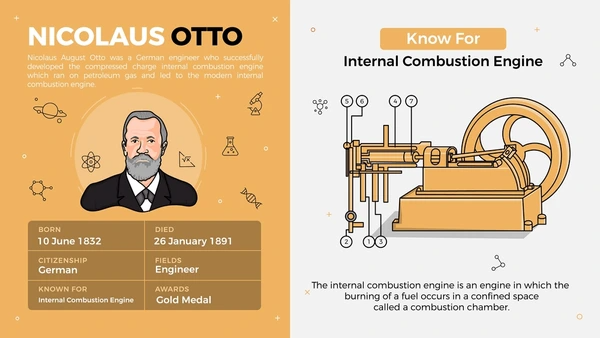
Components of An Otto Cycle
- Isentropic Compression (Process 1-2)
During this stage, the air-fuel mixture compresses without exchanging heat with the surroundings. As the gas volume decreases, its pressure and temperature rise significantly. This step is critical to the engine’s overall performance, as the compression ratio, or the ratio of initial to final volumes, directly influences thermal efficiency. Improving this ratio is key to optimizing energy output. - Isochoric Combustion (Process 2-3)
Here, the compressed air-fuel mixture ignites, typically via a spark plug, leading to a rapid rise in pressure while the volume stays constant. This stage adds heat to the system, causing a sharp increase in both pressure and temperature. The amount of heat added determines the engine’s power output, making this phase pivotal for maximizing performance. - Isentropic Expansion (Process 3-4)
The high-pressure gas expands without heat transfer, performing work by pushing the piston. During this expansion, the pressure and temperature drop while the volume increases. This step converts thermal energy into mechanical work, powering the engine. The expansion ratio, ideally equal to the compression ratio, significantly impacts energy conversion efficiency. - Isochoric Heat Rejection (Process 4-1)
In the final stage, the gas cools at a constant volume, reducing its pressure and temperature to the initial state. This step prepares the system for the next cycle by removing excess heat. Efficient heat rejection enhances the engine’s overall thermal performance and ensures smooth operation.
Thermodynamic Principles
The efficiency of this thermodynamic cycle stems from its governing processes. Thermal efficiency (η\eta) is calculated using the formula:
Here, r represents the compression ratio, while γ\gamma (gamma) is the specific heat ratio of the gas. Increasing the compression ratio significantly improves efficiency by maximizing the work extracted from the fuel. However, practical limitations such as engine knocking and material strength set upper limits to this ratio. Balancing these factors is crucial for achieving both optimal performance and durability in real-world applications.
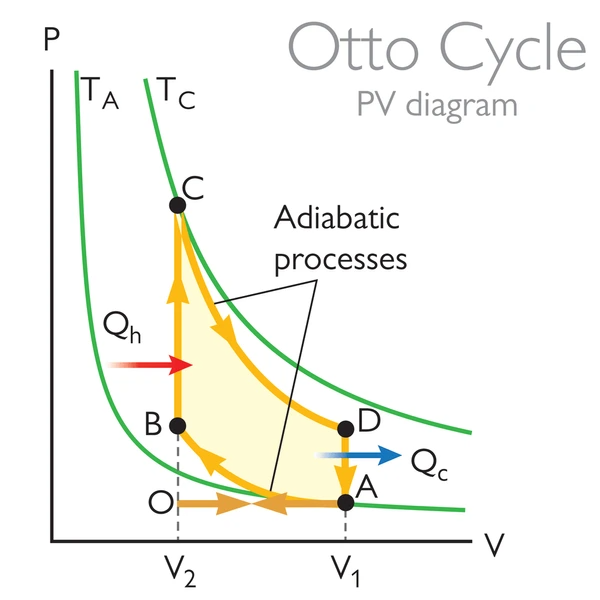
Advantages and Limitations of the Otto Cycle
Advantages
- Lower Emissions:
Engines based on this cycle produce fewer NOx and particulate emissions compared to diesel engines. They effectively utilize exhaust gas after-treatment systems, which are often less efficient in diesel engines. - Simpler Design and Operation:
These engines feature a straightforward design and use spark ignition for precise combustion control. This simplicity ensures smoother operation, easier maintenance, and overall reliability. - High Power Density:
The engines deliver impressive power outputs relative to their size, making them ideal for compact applications like automotive use and small-scale power generation. - Fuel Flexibility:
They can run on various fuels, including gasoline, alcohol, and their blends. This adaptability helps optimize performance and cost based on fuel availability.
Limitations
- Lower Thermal Efficiency:
Their thermal efficiency is generally lower than that of diesel engines due to the use of lower compression ratios to avoid knocking. - Knocking and Compression Limits:
Knocking remains a challenge, restricting the compression ratio and consequently limiting thermal efficiency and power output. - Pumping Losses:
At partial loads, these engines face pumping losses caused by throttling. This reduces efficiency as extra work is needed to overcome negative pressure differentials. - Heat and Friction Losses:
Heat dissipation and internal friction further reduce overall efficiency. These issues are heightened by high temperatures and pressures, leading to increased wear and maintenance demands.
Applications of Otto Cycle
- 1. Automotive Engines
Automotive engines widely use this thermodynamic cycle for reliable power and efficiency. Innovations like fuel injection and turbocharging enhance performance and fuel economy. - 2. Hybrid Systems
Hybrid powertrains combine combustion engines with electric motors, boosting efficiency. Hydrogen recovery and heat utilization further optimize eco-friendly vehicle performance. - 3. High-Performance Engines
Racing engines maximize power with technologies like variable valve timing, fuel injection, and high-octane fuels, delivering peak performance and precision. - 4. Combined Power Systems
Dual-fuel setups mix gasoline and water to reduce emissions and improve fuel economy, offering a more sustainable engine solution. - 5. Regenerative and Polytropic Expansion
Regenerative cycles control heat during expansion, improving thermal efficiency and lowering NOx emissions for cleaner, more efficient engines. - 6. Six-Stroke Engines
Six-stroke engines recover exhaust heat and convert it into usable work, significantly boosting efficiency with two extra power strokes. - 7. Dual-Fuel Engines
Flexible dual-fuel engines run on combinations like ammonia and methane, adapting seamlessly for industrial, marine, or other large-scale applications. - 8. Hydrogen Engines
Hydrogen-fueled engines deliver high power with low emissions. Turbocharged designs improve efficiency, advancing clean energy solutions for transportation.
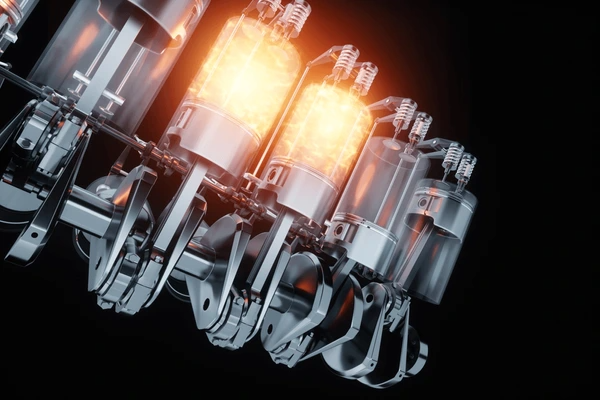
Application Cases
Product/Project | Technical Outcomes | Application Scenarios |
---|---|---|
Advanced Otto Cycle Engine | Higher efficiency with non-ideal gas models, especially in low-temperature regimes. | High-performance automotive engines requiring improved efficiency. |
Piston Ring for Otto Cycle Engines Mahle-Metal Leve SA | Enhanced durability and performance through laser surface heat treatment. | Automotive engines requiring high durability components. |
Supercharged Otto Cycle Engine GKN Aerospace (FFT) Ltd. | Improved power supply control with turbocharger and wastegate valve. | High-performance automotive and aerospace applications. |
Regenerative Otto Cycle Engine | Improved thermal efficiency and specific work with polytropic expansion. | Automotive engines aiming for higher efficiency and lower NOx emissions. |
Turbocharged PFI Hydrogen Engine | Higher power density and lower BSFC with Miller cycle integration. | Hydrogen-fueled automotive engines requiring high efficiency and low emissions. |
Latest Technical Innovations in Otto Cycle
- 1. Efficiency Improvements
Dynamic compression ratios optimize engine performance and reduce fuel consumption by up to 30%. Advanced ignition systems like laser and plasma ignition offer precise timing control, enabling leaner air-fuel mixtures for greater thermal efficiency and lower emissions. Turbocharging, combined with downsizing, enhances power while maintaining fuel efficiency, with modern turbos delivering improved boost control and responsiveness. - 2. New Materials and Technologies
Lightweight materials such as aluminum alloys and carbon composites reduce engine weight, boosting performance and efficiency. Low-friction coatings like diamond-like carbon minimize internal losses, enhancing durability and fuel economy. Advanced synthetic lubricants with high thermal stability further reduce wear, improving engine lifespan and efficiency. - 3. Design Modifications for Energy Savings
Adopting Atkinson cycle principles in modern engines increases thermal efficiency, especially in hybrid systems. Cylinder deactivation reduces fuel use during light loads without compromising performance. Efficient exhaust gas recirculation systems lower combustion temperatures, cutting NOx emissions while maintaining power output. - 4. Emission Reduction Technologies
Enhanced three-way catalysts improve pollutant conversion efficiency over a broader temperature range. Gasoline particulate filters capture fine particles, meeting stricter emission standards. Lean NOx traps reduce nitrogen oxides in lean-burn engines, offering cleaner exhaust profiles for modern combustion systems. - 5. Application of Advanced Control Systems
Model predictive control systems fine-tune engine operations in real-time, balancing efficiency and emissions. AI and machine learning technologies enable predictive maintenance and adaptive strategies, improving reliability and minimizing downtime by analyzing data from engine sensors.
To get detailed scientific explanations of Otto Cycle, try Patsnap Eureka.
