
What are Carbon Nanotubes?
Carbon nanotubes (CNTs) are rapidly transforming industries with their exceptional properties and wide-ranging applications. Renowned for their remarkable strength, lightweight design, and outstanding electrical conductivity, CNTs have become indispensable across diverse fields such as electronics and biomedicine. These cylindrical structures, meticulously formed from carbon atoms in a hexagonal lattice, are classified into single-walled (SWNTs) and multi-walled nanotubes (MWNTs). With diameters as small as 0.4 nanometers and lengths reaching micrometers, CNTs offer nearly boundless potential for advancing modern technology. Moreover, this article delves into their unique properties, explores their varied applications, and underscores their immense potential to revolutionize industries globally.
Types of Carbon Nanotubes
- Single-Walled Nanotubes (SWNTs): SWNTs are seamless cylinders made of a single graphene layer with diameters between 0.7 and 2 nanometers. Their unique chirality determines electrical behavior, making them either metallic or semiconducting. Highly flexible and strong, SWNTs are widely used in sensors, field-effect transistors (FETs), and conductive composites.
- Multi-Walled Nanotubes (MWNTs): MWNTs comprise multiple concentric graphene layers with diameters ranging from 2–100 nanometers. Their durable structure boosts strength, conductivity, and thermal stability, making them essential for supercapacitors, composites, and display technologies.
- Double-Walled Nanotubes (DWNTs): DWNTs combine two graphene cylinders, offering SWNT flexibility and MWNT durability. With excellent conductivity and strength, they excel in advanced composites and nanoelectronic applications.
- Y-Type Branched Nanotubes: Y-type nanotubes have branched structures formed during synthesis, offering distinct mechanical and electrical properties. Their unique geometry supports applications in high-performance composites and microelectronics by enhancing material networks.
- Chiral, Armchair, and Zigzag Nanotubes: These nanotubes differ in how graphene sheets are rolled. Armchair types are metallic, while zigzag and chiral types can be semiconducting or metallic. Their diverse electronic properties make them indispensable for sensors, nanoelectronics, and conductive materials.
Structure and Composition of Carbon Nanotubes
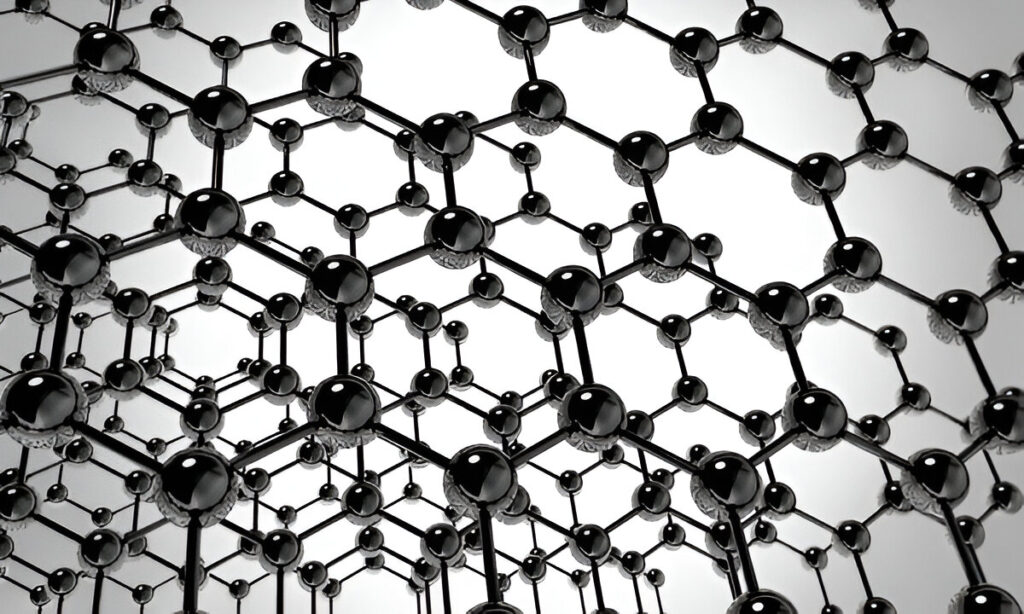
- Basic Structure
Carbon nanotubes (CNTs) are tubular structures with nanoscale diameters. They can be single-walled (SWCNTs) or multi-walled (MWCNTs) with concentric layers. Carbon atoms form a hexagonal lattice, similar to graphite, imparting exceptional mechanical, thermal, and electrical properties. The structure varies by chirality—armchair, zigzag, or chiral—which determines their electronic behavior. For instance, armchair CNTs are metallic, while zigzag and chiral types may exhibit metallic or semiconducting properties depending on their atomic arrangement. - Composition and Functionalization
The composition of CNTs can be tailored to enhance performance across various applications. By combining CNTs with organic or inorganic materials, composites gain improved strength, conductivity, and thermal stability. For example, reinforcing CNTs with other materials increases their tensile strength and Young’s modulus. Functionalizing CNTs with chemical groups like amino or hydroxyl groups enhances their dispersion and interaction with other substances, boosting stability and film-forming properties. This versatility expands CNTs’ applicability across multiple fields.
Properties of Carbon Nanotubes
- Outstanding Mechanical Strength
CNTs offer remarkable strength, with a Young’s modulus around 1 TPa and tensile strength reaching 150 GPa, far surpassing steel. Their unique cylindrical graphene structure provides exceptional toughness and elasticity, ideal for high-performance materials. - Superior Electrical Conductivity
Depending on chirality and diameter, CNTs can be metallic or semiconducting. SWCNTs show versatile electrical behaviors, while MWCNTs typically exhibit metallic conductivity. Their conductivity, up to 100 MA/cm², makes them indispensable for advanced electronics like transistors and nanoscale circuits. - Remarkable Thermal Performance
CNTs possess thermal conductivity exceeding 3000 W/m·K, outperforming diamond, with high stability at temperatures up to 2800°C in a vacuum. These properties arise from robust sp² carbon-carbon bonds, supporting applications in thermal management systems. - Versatile Chemical Reactivity
While chemically stable and corrosion-resistant, CNTs can be surface-functionalized to enhance compatibility with other materials or introduce new functionalities. This adaptability makes them valuable for sensors, catalysis, and drug delivery systems.
How Carbon Nanotubes Are Made
Chemical Vapor Deposition (CVD)
CVD is a widely used technique for synthesizing CNTs through the decomposition of hydrocarbon gases over metal catalysts like Fe, Ni, or Co.
- Catalyst Preparation: A substrate is coated with catalyst particles using methods like vacuum deposition or solution techniques.
- Growth Process: Carbon-containing gases (e.g., methane, acetylene) decompose at high temperatures (500-1000°C), allowing carbon atoms to diffuse and grow on the catalyst particles.
- Morphology Control: Adjusting catalyst size and reaction conditions influences the diameter, length, and type (SWCNTs or MWCNTs) of CNTs.
Arc Discharge Method
This method employs a high-temperature arc between graphite electrodes in an inert gas atmosphere, such as helium or argon, to synthesize CNTs.
- Arc Setup: Electric currents generate an arc that vaporizes the graphite, creating a carbon-rich environment.
- CNT Formation: Subsequently, carbon vapor condenses on cooler chamber surfaces, resulting in the production of multi-walled CNTs with excellent crystallinity.
- Purification: To ensure high-quality CNTs, impurities like amorphous carbon and metal particles are removed through acid treatment and filtration processes.
Laser Ablation
This process uses a high-power laser to vaporize a graphite target, producing CNTs as carbon atoms condense in the presence of catalysts like nickel or cobalt.
- Laser Process: A pulsed or continuous laser precisely vaporizes the graphite target embedded with catalysts, enabling controlled carbon release.
- Quality Control: Moreover, adjusting laser parameters, catalysts, and reaction conditions significantly influences the yield and purity, making this method ideal for producing high-quality single-walled CNTs.
High-Temperature Synthesis
Techniques such as self-propagating high-temperature synthesis (SHS) rely on exothermic reactions to generate heat, driving CNT formation.
- SHS Process: Reactants like carbonaceous materials and reducers initiate the reaction, producing CNTs with varying morphologies.
- Product Characteristics: This method yields multi-walled CNTs and carbon nanofibers, suitable for applications requiring diverse structural properties.
Low-Temperature Synthesis
CNTs can also form at lower temperatures using specific precursors and catalysts.
- Cellulose-Based Methods: Continuous oxidation processes at 240-400°C produce CNTs from natural wood fibers.
- Toluene Reduction: Using toluene and La/Ni catalysts, CNTs are synthesized at moderate temperatures around 350°C.
Mechanochemical Methods
Mechanical activation combined with chemical reactions facilitates CNT production.
- Activation Process: Amorphous carbon generated at 950°C is mechanically activated in a planetary mill to form multi-layer CNTs.
Applications of Carbon Nanotube
Engineering and Materials Science
CNTs continue to revolutionize composite materials by providing exceptional tensile strength and elasticity, making them indispensable for aerospace, automotive, and construction industries. These composites not only offer lightweight and durable solutions but also significantly enhance structural components’ performance. Additionally, CNT-based conductive composites play a critical role in antistatic coatings, EMI shielding, and adhesives, driving innovation in electronics and automotive applications.
Electronics and Electrical Engineering
CNTs are reshaping advanced display technologies, including field emission and vacuum fluorescent displays, due to their low-voltage electron emission and exceptional conductivity. In transistors, they surpass traditional silicon-based devices by offering higher mobility and operational frequencies. Furthermore, CNTs greatly enhance energy storage systems like lithium-ion batteries, supercapacitors, and fuel cells, boosting energy capacity and charge rates for a wide range of applications.
Biomedical Applications
SWCNTs are paving the way for advancements in drug delivery, effectively transporting genetic material and drugs, especially in cancer treatments and gene therapies. In tissue engineering, CNTs serve as robust scaffolds, aiding bone and cartilage repair. Moreover, their high sensitivity and specificity enable groundbreaking applications in biosensors for medical diagnostics and environmental monitoring.
Environmental Applications
CNTs excel in adsorbing heavy metals and organic pollutants, making them ideal for water purification and air filtration systems. In energy systems, CNTs enhance the performance of fuel cells and hydrogen storage solutions, driving progress in sustainable energy technologies and reducing environmental impact.
Industrial and Mechanical Uses
CNTs play a pivotal role in the development of sensors and actuators for industrial automation and control systems. Their unmatched strength and durability significantly improve materials used in sports equipment, automotive parts, and aerospace components, offering an optimal balance between weight and performance to meet industry demands.
Emerging Innovations
CNTs are unlocking new possibilities in neurobiology by promoting neural cell growth and supporting brain-computer interface development. Additionally, in solar energy, CNTs enhance photovoltaic efficiency by improving light absorption and charge transport, paving the way for more cost-effective and sustainable solar power solutions.
Application Cases
Product/Project | Technical Outcomes | Application Scenarios |
---|---|---|
Carbon Nanotube Fibers | Excellent mechanical and electrical properties, used as gas adsorbents, templates, actuators, composite reinforcements, catalyst supports, probes, chemical sensors, nano-reactors. | Aerospace, energy, medicine, and chemical industry. |
Neural Cell Growth Substrates | Small size, flexibility, strength, inertness, electrical conductivity, and ease of modification with biological compounds. | Neuroscience research, neural network organization studies, neuron monitoring or stimulation. |
Anti-curl Back Coating | Improved electrical and mechanical properties, addressing issues of carbon nanotube solubility and resistivity range. | Electronics, particularly in applications requiring stable and durable coatings. |
Carbon Nanotube Orientation Detection | Accurate detection of carbon nanotube orientation using laser beams, enhancing the precision of nanotube applications. | Microelectronics, nanotechnology research, and development. |
Carbon Nanotube-Metal Oxide Composite | Uniform dispersion of metal oxide particles in carbon nanotubes, enhancing electrical and mechanical properties. | Advanced materials for electronics, energy storage, and catalysis. |
To get detailed scientific explanations of Carbon Nanotube, try Patsnap Eureka.
