
What are Ionic Compounds?
Ionic compounds are essential chemical compounds formed by the interaction of positively charged cations and negatively charged anions. Created through the transfer of electrons from metals to non-metals, these compounds are bonded by strong electrostatic forces known as ionic bonds. Represented by the general formula Mm+Xn-, where Mm+ denotes the metal cation and Xn- the non-metal anion, ionic compounds play a vital role in numerous chemical and industrial applications. This article explores their formation, properties, and significance in various fields.
Structural Characteristics of Ionic Compounds
- Lattice Structure: Ionic compounds form a lattice structure where each ion is surrounded by oppositely charged ions. This arrangement maximizes electrostatic attraction and minimizes repulsion, contributing to the stability of the compound.
- Coordination Number: This refers to the number of oppositely charged ions surrounding a given ion. For example, in sodium chloride (NaCl), each Na+ ion is surrounded by six Cl- ions, giving it a coordination number of six.
- Unit Cell: The smallest repeating unit in the lattice structure is known as the unit cell. The geometry of the unit cell (e.g., cubic, tetragonal) defines the overall symmetry and properties of the crystal.
Key Properties of Ionic Compounds
- Structural and Physical Properties: Crystalline solids with high melting and boiling points characterize these compounds due to strong electrostatic forces in their ordered lattices. They are typically hard, brittle, and dense, thanks to the close packing of ions in the crystal structure.
- Electrical Conductivity: In solid form, these materials poorly conduct electricity since their ions are immobile. However, when melted or dissolved, the free-moving ions enable effective electrical conductivity, making them ideal for use in electrolytes for batteries and fuel cells.
- Solubility: Most dissolve well in polar solvents like water, driven by interactions between ions and polar molecules. This property is vital in industrial applications such as purifying hydrocarbons and producing low-sulfur fuels.
- Thermal Stability: Their strong ionic bonds grant them excellent thermal stability, making them suitable for high-temperature uses like ceramics and refractory materials. Additionally, ionic liquids, a specialized subset, offer stability at relatively low temperatures for niche applications.
- Low Vapor Pressure: With exceptionally low vapor pressures, especially in ionic liquids, these compounds serve as eco-friendly alternatives to volatile organic compounds. This property reduces air pollution risks and enhances safety in green chemical processes.
- Chemical Versatility: Their properties are highly tunable by altering the ions involved, enabling the design of materials tailored for specific needs. For example, ionic liquids can be engineered for specific viscosities, conductivities, or solubilities, making them versatile in catalysis, electrochemistry, and separation technologies.
Understanding Chemical Bonding in Ionic Compounds
- Ionic Bond Formation
Ionic bonds form when a metal transfers electrons to a non-metal, creating positively charged cations and negatively charged anions. This electron exchange results in a strong electrostatic attraction between the opposite charges, which defines ionic bonding. - Lattice Energy
Lattice energy, the energy released during the formation of a crystalline lattice, greatly impacts the stability of ionic compounds. Its magnitude depends on the ion charges and their separation distance, as described by Coulomb’s Law. - Electrostatic Interactions
Electrostatic forces govern the properties of ionic compounds, influencing their potential energy and physical characteristics. These interactions contribute to ionic compounds’ high melting and boiling points, making them uniquely robust.
How Ionic Compounds Form: Electron Transfer Mechanisms
- a. Ionization of Metal Atoms: When a metal atom loses one or more electrons, it becomes a positively charged ion (cation). For example, a sodium atom (Na) loses one electron to form a sodium ion (Na+): [ \text{Na} \rightarrow \text{Na}^+ + e^- ]
- b. Electron Acceptance by Non-Metal Atoms: A non-metal atom gains the electron(s) lost by the metal atom, becoming a negatively charged ion (anion). For instance, a chlorine atom (Cl) gains one electron to form a chloride ion (Cl-): [ \text{Cl} + e^- \rightarrow \text{Cl}^- ]
- c. Formation of Ionic Bond: The electrostatic attraction between the Na+ and Cl- ions results in the formation of sodium chloride (NaCl), an ionic compound: [ \text{Na}^+ + \text{Cl}^- \rightarrow \text{NaCl} ]
Influence of Different Elements on Electron Transfer
The efficiency and nature of electron transfer can vary depending on the elements involved. Factors such as ionization energy, electron affinity, and atomic size play crucial roles.
- a. Ionization Energy: Elements with lower ionization energies (e.g., alkali metals) readily lose electrons, facilitating the formation of cations.
- b. Electron Affinity: Elements with higher electron affinities (e.g., halogens) are more likely to gain electrons, forming stable anions.
- c. Atomic Size: Smaller atoms tend to have higher ionization energies and electron affinities, influencing the ease of electron transfer.
Common Examples of Ionic Compounds
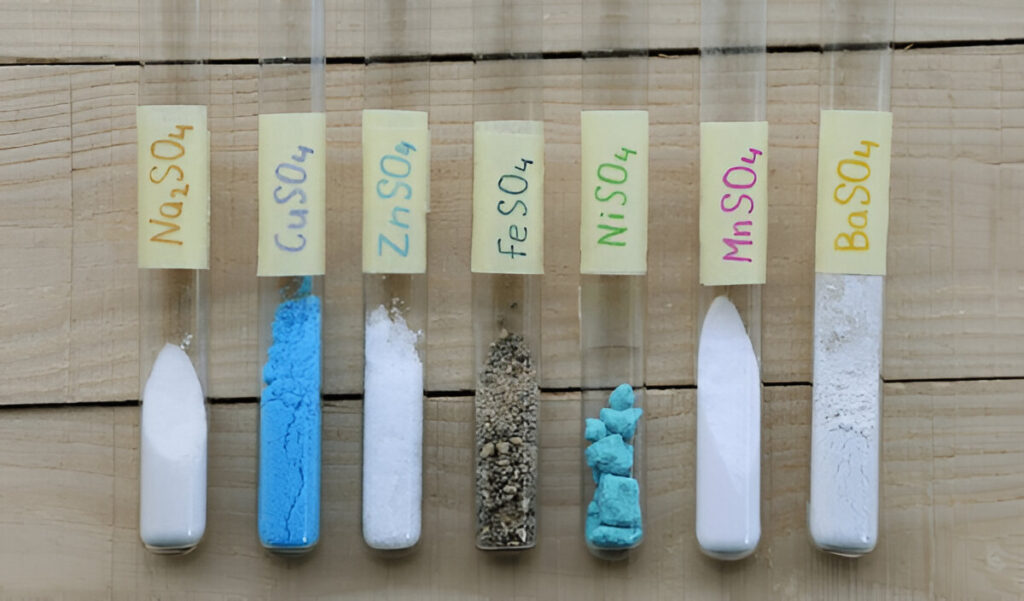
- Sodium Chloride (NaCl): Commonly known as table salt, NaCl combines sodium cations (Na⁺) and chloride anions (Cl⁻). Widely used for seasoning, preservation, and road de-icing, its high solubility in water and conductivity in molten form make it vital for various industries.
- Calcium Carbonate (CaCO₃): Found in limestone, marble, and chalk, CaCO₃ consists of calcium cations (Ca²⁺) and carbonate anions (CO₃²⁻). It’s essential in construction for cement and lime, plastics as a filler, and pharmaceuticals as a supplement or antacid.
- Potassium Nitrate (KNO₃): Known as saltpeter, KNO₃ comprises potassium cations (K⁺) and nitrate anions (NO₃⁻). It plays a key role in fertilizers, food preservation, and as an oxidizer in fireworks and gunpowder, supporting agriculture with its nitrogen content.
- Magnesium Oxide (MgO): Composed of magnesium cations (Mg²⁺) and oxide anions (O²⁻), MgO is a refractory material for furnace linings, an electrical insulator, and a dietary magnesium supplement. Its high thermal stability suits it for demanding applications.
- Ammonium Chloride (NH₄Cl): NH₄Cl features ammonium cations (NH₄⁺) and chloride anions (Cl⁻). It’s used in batteries, as a flux in metalwork, and as a nitrogen source in fertilizers. Its versatility extends to textiles and pharmaceuticals.
- Calcium Sulfate (CaSO₄): Known as gypsum, CaSO₄ combines calcium cations (Ca²⁺) and sulfate anions (SO₄²⁻). It’s vital in plaster, drywall, soil conditioning, and medical casts, valued for its durability and hardness.
- Aluminum Oxide (Al₂O₃): Al₂O₃, or alumina, features aluminum cations (Al³⁺) and oxide anions (O²⁻). It serves as an abrasive, furnace lining material, and catalyst. Its hardness and stability make it indispensable in industrial applications.
- Barium Sulfate (BaSO₄): Composed of barium cations (Ba²⁺) and sulfate anions (SO₄²⁻), BaSO₄ excels as a contrast agent in medical imaging, an additive in drilling fluids, and a component in paints. Its density and X-ray opacity make it invaluable for diagnostics.
Applications of Ionic Compounds
1. Industrial Applications
Ionic liquids play a key role as process aids in chemical manufacturing. They support hydrosilylation for organosilicon synthesis, enabling catalyst immobilization, recovery, and reuse. Additionally, they serve as solvents and catalysts in reactions like alkylation, polymerization, and metathesis. For instance, BMP and BMIM chloroaluminate are widely used in alkylation processes.
2. Pharmaceutical and Medical Applications
Ionic liquids are valuable in synthesizing, extracting, and purifying bioactive compounds. They act as green solvents and catalysts, stabilizing reactive intermediates while facilitating catalyst reuse. Some ionic liquids also function as pharmaceutical ingredients due to their favorable properties, supporting sustainable practices in drug manufacturing.
3. Environmental Applications
Ionic liquids contribute to eco-friendly solutions, including removing sulfur, nitrogen, and metals from fuels, aiding low-sulfur fuel production. They are also used in CO₂ capture, wastewater treatment, SO₂ absorption, and plastic recycling, enhancing waste management and pollution control.
4. Energy Storage and Electrochemical Devices
Ionic compounds are critical in batteries, fuel cells, and capacitors, acting as electrolytes and enabling ionic conduction. For example, lithium perchlorate and LiPF6 are common electrolytes in lithium-ion batteries, improving energy storage and efficiency in electrochemical devices.
5. Advanced Materials and Composites
Ionic liquids enhance polymer composites by acting as solvents, plasticizers, and coupling agents. They improve aerogels, films, and biocomposites, offering environmentally friendly options for advanced material production across industries.
6. Energetic Materials
Energetic ionic compounds, including salts and ionic liquids, are vital in explosives, propellants, and gas agents. These compounds deliver high energy with low sensitivity and are designed for thermal stability and environmental safety.
7. Liquid Crystals and Electronics
Ionic liquid crystals enhance stability and performance in optical and electronic applications. They are used in displays, optical films, and semiconductors, supporting innovation in electronics, diagnostics, and security technologies.
Application Cases
Product/Project | Technical Outcomes | Application Scenarios |
---|---|---|
Ionic Liquid Regeneration Process | Efficient separation and recovery of cationic and anionic components from deactivated ionic liquids, enabling reuse and reducing waste. | Chemical processing industries where ionic liquids are used as catalysts or solvents. |
Ionic Fluids | Preparation of ionic compounds that remain liquid or semi-solid at temperatures below 150°C, providing stable and conductive properties. | Electrochemical devices and applications requiring stable, low-temperature ionic fluids. |
Ionic Mesogenic Compounds | Use in liquid crystal media and devices, providing excellent electrooptical and charge transport properties. | Optical and electronic devices, including displays, electrochemical cells, and security markings. |
Ionic Compound for Capacitors | Excellent electrochemical stability and ionic conductivity, enhancing capacitor performance. | Capacitor manufacturing and applications requiring high stability and conductivity. |
Room Temperature Ionic Liquids | Hydrophobic, conductive, and stable ionic liquids formed by mixing neutral organic ligands with metal salts. | Solvents and electrochemical devices requiring stable and conductive ionic liquids. |
Latest Technical Innovations in Ionic Compounds
Innovative Synthesis Techniques
Advancements in ionic compound synthesis have produced materials with enhanced properties. For example, perfluorosulfonimide-based compounds now offer better acidity, solubility, and dissociation. Their delocalized anionic charge ensures stability, even in protonated form, making them ideal for catalysis and electrochemistry. The precise chemical reactions behind these innovations optimize their functionality for demanding applications.
Improved Properties and Versatility
Recent developments have significantly enhanced the performance of ionic compounds. These include improved stability, superior solubility, and better dissociation characteristics, making them more reliable under varied conditions. Polymerized ionic liquids (PILs) combine the advantages of liquids and polymers, excelling in applications like drug delivery and catalytic processes while improving immobilization efficiency.
Expanding Practical Applications
Innovations in ionic liquids (ILs) have expanded their roles beyond solvents to vital tools in reactions and extractions. Synthesized ILs now serve as effective embedding materials and catalysts, excelling in applications like drug delivery systems with controlled release and improved stability. In catalysis, these compounds enhance activity while enabling easy recovery and reuse, streamlining industrial processes.
To get detailed scientific explanations of Ionic Compounds, try Patsnap Eureka.
