
What Is a Decoupling Capacitor?
A decoupling capacitor plays a vital role in electronic circuits by stabilizing power supply voltages and reducing noise. Acting as a local reservoir of charge helps prevent voltage fluctuations caused by switching activities or other circuit components. Decoupling capacitors ensure smooth operation by decoupling different parts of a circuit, making them indispensable in maintaining reliable performance and minimizing electrical interference. In this article, we’ll explore what a decoupling capacitor is, how it works, and why it’s so important in electronics.
How Decoupling Capacitors Work
Fundamental Principle
Capacitors play a crucial role in stabilizing power supply voltages and suppressing noise in electronic circuits. They act as local energy reservoirs, supplying instantaneous current to components during transient fluctuations or sudden demands. This prevents voltage dips and noise from disrupting sensitive parts of the circuit.
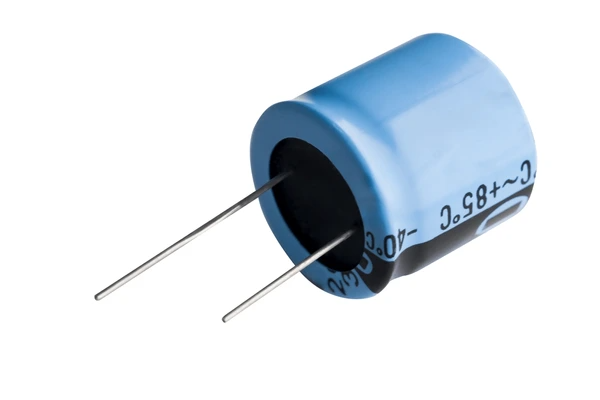
Design and Operation
1. Strategic Placement
- Capacitors are placed parallel to circuit components they support.
- Positioning them close to the components minimizes parasitic inductance and enhances effectiveness.
2. Charging Process
- Under normal conditions, capacitors draw energy from the power supply to charge.
- This readiness ensures they can respond immediately to changes in demand.
3. Discharging for Stability
- During sudden current demands, capacitors release stored energy to stabilize voltage.
- This discharge prevents voltage drops that could disrupt circuit performance.
4. Recharging Cycle
- After supplying energy, capacitors recharge to prepare for future fluctuations.
- This cyclical process maintains consistent power delivery and system stability.
How to Use Decoupling Capacitors Effectively
Selecting Capacitors for Optimal Performance
- Use multiple capacitors with varying capacitance values and self-resonant frequencies to achieve broad impedance coverage.
- This combination ensures effective suppression of noise across a wide range of frequencies.
Strategic Distribution of Components
- Distribute capacitors among powered components in organized clusters for efficient energy delivery.
- Incorporate individual cells containing capacitors and current-limiting resistors to enhance stability.
Optimizing Placement for Efficiency
- Utilize effective radii models to position capacitors within optimal charging and discharging distances from their loads.
- Proper placement reduces delays and ensures efficient energy transfer during transient demands.
High-Density On-Chip Capacitors
- Allocate on-chip capacitors in unused white space or embed them within standard cell rows for maximum density.
- This approach saves space while maintaining reliable power delivery in compact designs.
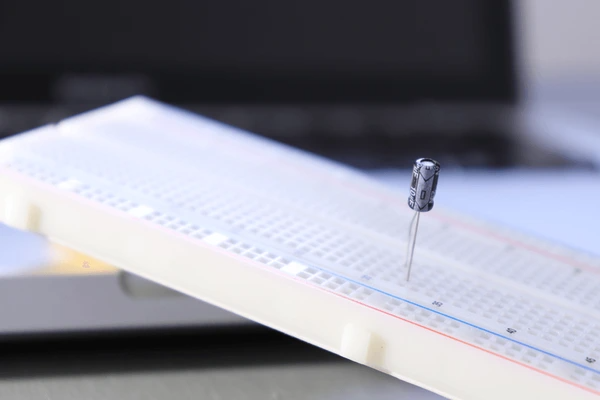
Key Benefits of Using Decoupling Capacitors
1. Noise Reduction and Power Stability
- Capacitors play a critical role in minimizing electrical noise and maintaining power stability in high-speed circuits.
- By offering a low-impedance path, they divert transient currents and filter out high-frequency noise.
- This helps prevent issues like ground bounce, power droop, and switching noise, ensuring signal integrity.
2. Instantaneous Charge Supply
- Acting as local charge reservoirs, capacitors provide immediate current during load changes and switching events.
- This stabilizes supply voltage, avoiding voltage dips or spikes that could disrupt circuit performance.
- By isolating the load from the power source, they ensure smooth operation during high-demand events.
3. Effective High-Frequency Bypassing
- At high frequencies, network inductance can degrade power delivery.
- Capacitors create a low-impedance bypass for high-frequency currents, improving power delivery efficiency.
- This feature is essential for modern circuits running at multi-gigahertz frequencies.
4. Strategic Placement for Optimization
- Proper placement near the load minimizes parasitic inductance and enhances performance.
- Distributed capacitor networks, designed based on power grid impedance, provide efficient noise suppression.
- Advanced techniques like genetic algorithms help optimize capacitor values and locations for better results.
Applications of Decoupling Capacitors in Circuit Design
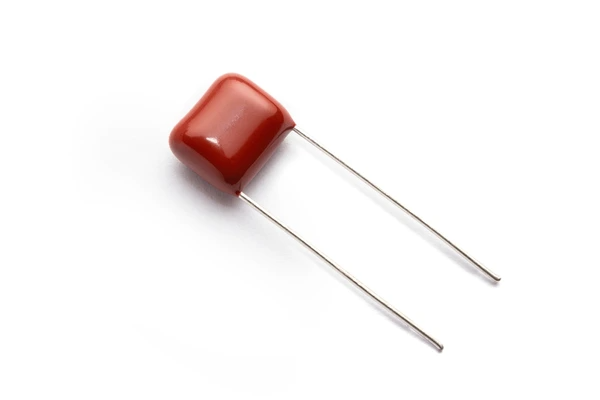
Fundamentals and Functionality
- Capacitors help isolate sections of a circuit, reducing noise and voltage fluctuations.
- Acting as local charge reserves, they smooth out voltage variations during switching events.
- Placed close to power-consuming circuits, they minimize parasitic inductances and resistances for better efficiency.
- For high-frequency gigahertz circuits, on-chip options like MIM (metal-insulator-metal) and deep trench capacitors are essential.
Placement and Configuration Strategies
- Distribute capacitors in clusters near powered components for optimal noise suppression.
- Each cluster often includes parallel capacitor cells with series resistors to manage current during defects.
- Placement must consider effective radii to ensure capacitors function efficiently within their impedance range.
- Additional mounting pads can support selective tuning and enhance circuit characteristics post-fabrication.
Design and Noise Suppression Techniques
- Optimizing factors like capacitance value, type, and self-resonant frequency is key to achieving a desired impedance profile.
- Advanced methods like circuit optimizations and remote capacitor designs increase density without extra process steps.
- Proper arrangement on PCBs ensures effective suppression of ΔI noise and maintains power integrity, especially at high frequencies.
Choosing the Right Decoupling Capacitor for Your Circuit
1. Define Circuit Requirements
- Frequency Range: High-speed circuits demand capacitors with low self-inductance, such as ceramic capacitors.
- Voltage Stability: Consider the circuit’s operating voltage and fluctuations to ensure the capacitor’s rated voltage exceeds the maximum voltage in the system.
2. Choose the Appropriate Type
- Ceramic Capacitors: Ideal for high-frequency applications due to low equivalent series resistance (ESR) and inductance.
- Electrolytic Capacitors: Suitable for low-frequency, high-capacitance needs like power filtering.
- Polymer Capacitors: Excellent for stable performance over a wide temperature range.
3. Consider Capacitance and Tolerance
- Capacitance Value: Select based on the impedance required to handle noise at different frequencies.
- Tolerance: Choose a tight tolerance for precise filtering or a wider tolerance for general noise suppression.
4. Optimize Placement
- Position capacitors close to power-consuming components to minimize parasitic inductances.
- Use a mix of high-capacitance and high-frequency capacitors in parallel to handle a broader noise spectrum.
5. Account for Self-Resonant Frequency
- Match the capacitor’s self-resonant frequency to the noise frequency for effective suppression.
- Use multiple capacitors with varying self-resonant frequencies for broadband noise reduction.
6. Plan for Future Adjustments
- Include extra mounting pads for post-fabrication tuning.
- Opt for modular designs to allow capacitor upgrades or replacements as circuit demands evolve.
To get detailed scientific explanations of Decoupling Capacitors, try Patsnap Eureka.
