
Lanthanide contraction is a subtle but significant trend in the periodic table, particularly across the lanthanide series. It refers to the gradual decrease in atomic and ionic radii of the lanthanide elements from lanthanum (La) to lutetium (Lu), despite an increasing atomic number. This contraction influences the chemistry of not just the lanthanides, but also elements in adjacent groups and periods. This article explores the causes, consequences, and real-world examples of lanthanide contraction, helping students and professionals understand its critical role in inorganic chemistry.
What Is Lanthanide Contraction?
What is lanthanide contraction? Eureka Technical Q&A explains that lanthanide contraction is the gradual decrease in atomic and ionic radii across the lanthanide series, caused by poor shielding of nuclear charge—affecting the chemistry of elements that follow in the periodic table.
Lanthanide contraction is the term used to describe the steady decrease in the size of trivalent lanthanide ions (Ln³⁺) across the series from La to Lu. Even though each element has an increasing number of electrons and protons, the radius does not increase—in fact, it decreases slightly with each step.
This phenomenon is observed in both atomic and ionic radii, especially in ions with a +3 charge, which are the most stable oxidation state of lanthanides.
Causes of Lanthanide Contraction
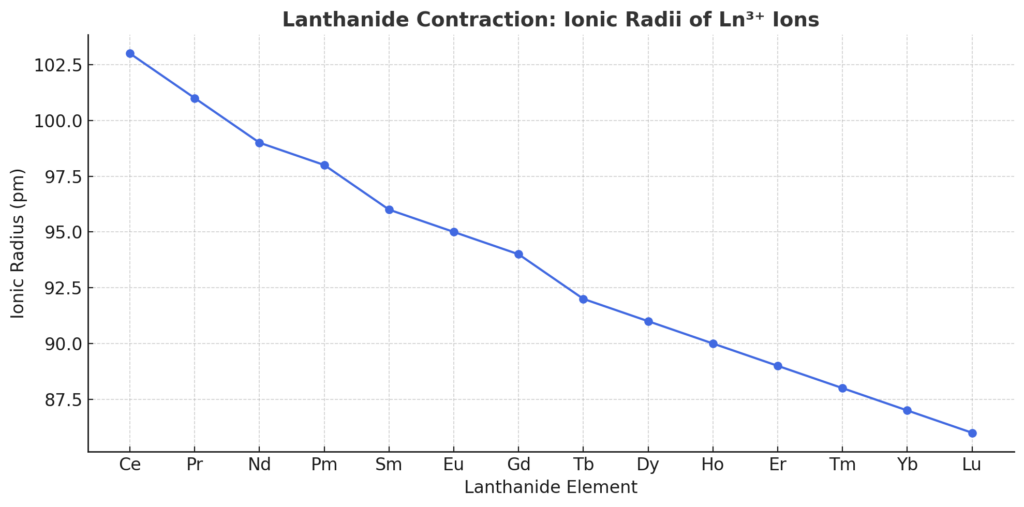
The primary reason behind lanthanide contraction lies in poor shielding by 4f electrons.
1. Poor Shielding Effect of 4f Orbitals
As electrons are added to the 4f subshell, they do not effectively shield the increased nuclear charge. As a result, the effective nuclear charge increases, pulling electrons closer to the nucleus and leading to a smaller atomic or ionic size.
2. Increasing Nuclear Charge
With each step across the lanthanide series, the number of protons increases by one. The growing positive charge attracts electrons more strongly, adding to the contraction.
3. Constant Addition to Inner Orbitals
The added electrons go into inner 4f orbitals rather than outer shells, so the outermost electron cloud remains roughly the same but is drawn closer to the nucleus over time.
Observed Trends in Lanthanide Radii
Element | Ionic Radius (Ln³⁺, pm) |
---|---|
La | 106 |
Ce | 103 |
Nd | 98 |
Gd | 93.8 |
Lu | 86 |
From La to Lu, the ionic radius decreases by nearly 20 pm, a significant shift for chemical bonding and reactivity.
Effects of Lanthanide Contraction
Lanthanide contraction affects the chemical and physical properties of not only the lanthanides but also the transition elements that follow.
- Atomic and Ionic Size Reduction: The most direct effect is the decrease in atomic and ionic radii as one moves down the lanthanide series .
- Structural Variations in Coordination Complexes: The contraction leads to significant structural differences in coordination polymers and complexes. For instance, the coordination number and geometry can vary depending on the lanthanide ion involved. Lutetium often forms unique complexes with a coordination number of 9 compared to the 10-coordinated lanthanides like lanthanum and neodymium.
- Luminescent Properties: The contraction affects the luminescent properties of lanthanide compounds. Varying the ratio of lanthanides in the reaction mixture allows researchers to obtain near-white-light-emitting samples, highlighting how f-block contraction tunes luminescent properties.
- Selective Cocrystallization and Separation: The contraction effect influences selective cocrystallization and separation strategies for lanthanide ions, as seen in the formation of cocrystals with tetra-2-pyridinylpyrazine.
- Impact on Material Properties: The contraction impacts the design and functionality of materials such as metal-organic frameworks (MOFs) and coordination polymers, affecting their applications in gas storage, catalysis, and sensing.
Real-World Examples
Lanthanide Coordination Polymers
Researchers synthesized coordination polymers using cyclohexanocucurbituril ligands and various lanthanides. Each lanthanide produced a distinct crystal structure. The f-block contraction reduced ionic radii across the series, leading to clear structural variations between early and late lanthanides.
Metal-Organic Frameworks (MOFs) with 2,5-Pyrazinedicarboxylic Acid
Lanthanide contraction significantly altered the architecture of MOFs built with 2,5-pyrazinedicarboxylic acid. Early lanthanides formed one structural type, while later lanthanides formed another. These variations resulted in mixed-metal MOFs with unique and tunable luminescent properties.
Phenanthroline-Based Lanthanide Complexes
Complexes with phenanthroline ligands clearly reflected the contraction effect. Lutetium formed a 9-coordinated complex, unlike lanthanum, neodymium, and europium, which all maintained 10 coordination sites. This shift highlighted the impact of decreasing ionic radii on coordination geometry.
Porous Frameworks for CO₂ Capture
Porous frameworks using carborane–Ln₂(CO₂)₆ units showed contraction-induced changes in framework topology. The reduced lanthanide size improved CO₂ selectivity by influencing pore size and framework rigidity. These structural differences enhanced performance in gas separation and environmental applications.
Application Cases
Product/Project | Technical Outcomes | Application Scenarios |
---|---|---|
Lanthanide MOFs Novosibirsk State University | Formation of new MOFs with wide color range luminescence spectra influenced by lanthanide contraction | Light-emitting materials and color tuning applications |
Lanthanide Coordination Polymers Xiamen University | Structural variations attributed to lanthanide contraction effect in hydrothermal synthesis | Materials science and crystal engineering |
2D Lanthanide Coordination Polymers South China Normal University | Different ABAB stacking and hydrogen bonding interactions due to lanthanide contraction | Solid-state luminescent materials and sensors |
Phenanthroline Lanthanide Complexes Moscow State University Lomonosov | Unique 9-coordinated lutetium complex formation due to lanthanide contraction | Coordination chemistry and structural studies of f-elements |
FAQs
It explains similarities between transition elements, affects complex formation, and influences the design of functional materials and metal separation techniques.
The 4f electrons, which provide poor shielding against the increasing nuclear charge, are primarily responsible.
No. It also impacts the properties of post-lanthanide transition metals (like Hf) and elements in nearby groups.
It causes deviations in atomic size and ionization energies, especially when comparing elements across periods in the d-block.
Yes, using techniques like X-ray crystallography, spectroscopy, or by observing shifts in bond lengths and ionic radii.
Conclusion
Lanthanide contraction is a small but powerful trend that influences atomic size, reactivity, and chemical behavior across the periodic table. It is caused by the poor shielding of 4f electrons, leading to a steady reduction in ionic and atomic radii across the lanthanide series. The consequences are far-reaching, affecting transition metal chemistry, material properties, and separation technologies. Understanding this phenomenon provides deeper insight into the structure and periodicity of the elements.
How PatSnap Eureka Accelerates Research on Lanthanide Contraction
Lanthanide contraction is a critical concept in inorganic and materials chemistry, influencing trends in coordination chemistry, ionic radii, and electronic properties across rare earth elements. PatSnap Eureka equips researchers with deep, data-driven insights to explore how this phenomenon is being applied and advanced globally.
- Patent Intelligence: Eureka scans global patent databases to uncover innovations related to lanthanide contraction, including its impact on compound design, separation techniques, and advanced material development.
- Competitive Landscape Tracking: Identify how academic institutions and industry leaders are leveraging 4f orbital contraction in areas like catalysis, magnetic materials, and optical devices—revealing strategic research directions.
- Trend Forecasting: With AI-powered analytics, Eureka maps emerging applications of 4f orbital contraction in energy storage, electronics, and rare-earth recycling, helping guide forward-looking R&D.
- Technical Clustering: Visual clustering tools highlight active research zones where 4f orbital contraction plays a central role, such as in ligand field design, nanoparticle synthesis, and functional material innovation.
By turning complex patent data into clear, actionable insight, Eureka accelerates innovation in fields where lanthanide contraction drives unique material behavior and performance.
To get detailed scientific explanations of Lanthanide Contraction, try Patsnap Eureka.
