
Introduction: Longitudinal Wave
A longitudinal wave is a type of wave where particles in the medium move parallel to the wave’s direction of propagation. Unlike transverse waves, which involve perpendicular motion, longitudinal waves create regions of compression and rarefaction as they move. These waves are essential in nature and technology, from sound waves traveling through air to seismic P-waves during earthquakes.
In this guide, we’ll explore the properties, examples, and applications of longitudinal waves to help you understand their importance in physics and real-world scenarios.
What is a longitudinal wave? Eureka Technical Q&A explains how particles move back and forth in the same direction as the wave travels—like sound waves—making it easy to understand this fundamental wave type.
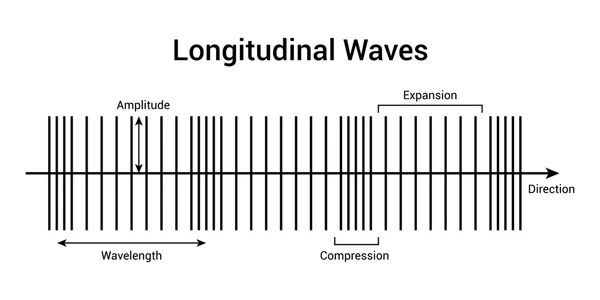
Key Properties of Longitudinal Waves
- Direction of Particle Displacement: In longitudinal waves, the particles of the medium oscillate back and forth in the same direction as the wave travels. This is in contrast to transverse waves, where particle displacement is perpendicular to the direction of wave propagation.
- Compression and Rarefaction: Longitudinal waves, also called compression waves, create regions of compression and rarefaction as they travel through a medium. During compression, particles push closer together, increasing pressure. In rarefaction, particles spread apart, decreasing pressure.
- Speed of Propagation: The speed of longitudinal waves varies based on the medium. In solids, they travel faster than in liquids or gases due to the closer proximity of particles in solids.
- Wavefront Characteristics: In a longitudinal wave, the wavefront is a plane normal to the direction of propagation. The amplitude of the wave remains constant during propagation until it interacts with another wave or medium.
- Energy Transfer: Longitudinal waves transfer energy through particle oscillations along the wave’s direction. This energy moves as a series of compressions and rarefactions within the medium.
How Longitudinal Waves Work
The working mechanism of longitudinal waves involves the transfer of energy through the medium via particle interactions. When a longitudinal wave travels through a medium, it creates alternating regions of compression (high pressure) and rarefaction (low pressure). The particles in the medium move back and forth in the direction of the wave, transferring energy from one particle to the next. This process continues, allowing the wave to propagate through the medium.
The speed of longitudinal waves depends on the medium’s properties, such as density and elasticity. For instance, in solids, the wave speed is influenced by the material’s stiffness and density, while in liquids and gases, it is affected by compressibility and density.
Factors Affecting Longitudinal Wave Speed
- Temperature: Temperature affects the medium’s density and elasticity. For gases, increasing the temperature reduces density and enhances elasticity, leading to faster wave speed.
- Medium Composition and Structure: Internal structures, such as cracks, inclusions, or variations in cross-sections, alter wave propagation. For example, in cracked porous solids, crack characteristics impact phase velocity and wave attenuation.
- External Constraints and Friction: Constraints like friction or external loads in cylindrical bodies modify wave parameters. Numerical studies show that these factors can increase stresses and particle velocities, affecting wave behavior over time.
- Material Anisotropy: In anisotropic materials, wave speed varies based on the propagation direction relative to crystallographic axes. For instance, ultrasonic surface skimming longitudinal waves exhibit direction-dependent speeds, measurable through time-of-flight analysis.
Advantages and Disadvantages of Longitudinal Waves
Advantages of Longitudinal Waves
- Efficient Energy Transfer: Longitudinal waves are highly efficient in transferring energy through a medium, making them ideal for applications such as acoustic communication and power transmission in sealed environments.
- Directional Propagation: These waves can be directed and focused more easily compared to transverse waves, which is advantageous in applications requiring precise wave guidance and control.
- Versatility in Different Media: Longitudinal waves can propagate through solids, liquids, and gases, making them versatile for various industrial and scientific applications, including non-destructive testing and medical ultrasonography.
Disadvantages of Longitudinal Waves
- Sensitivity to Medium Properties: The propagation of longitudinal waves is highly dependent on the medium’s properties, such as density and elasticity, which can vary significantly in heterogeneous or multiphase materials, leading to complex wave behavior and potential signal distortion.
- Attenuation and Dispersion: Longitudinal waves can experience significant attenuation and dispersion, especially in media with high viscosity or complex internal structures. This can limit the effective range and resolution of wave-based sensing and communication systems.
- Complexity in Analysis and Modeling: The mathematical modeling and analysis of longitudinal wave propagation can be complex, requiring advanced computational techniques and software to solve the governing equations accurately. This complexity can pose challenges in designing and optimizing systems that rely on longitudinal wave propagation.
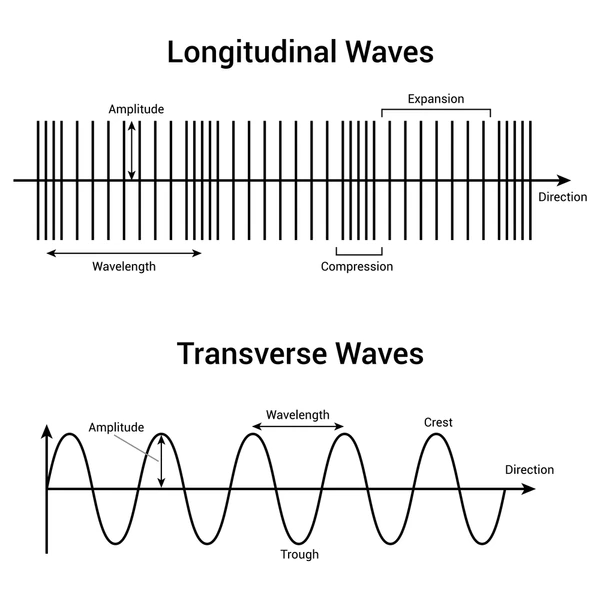
Longitudinal Wave vs. Transverse Wave
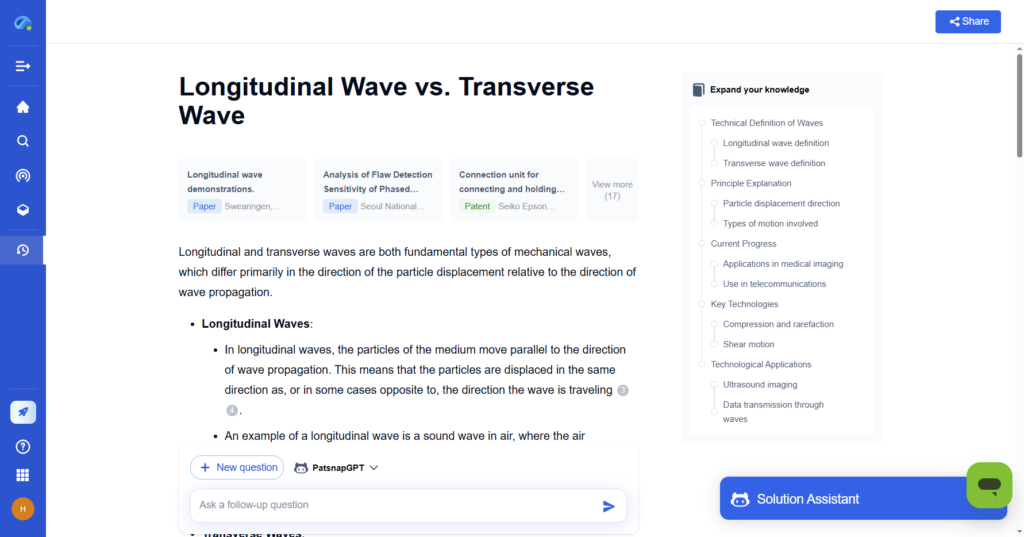
Propagation Mechanisms
- Longitudinal Waves: In longitudinal waves, the particle displacement is parallel to the direction of wave propagation. These waves involve compressions and rarefactions as they travel through the medium, making them also known as compression waves. They can propagate through solids, liquids, and gases. The speed of longitudinal waves depends on the medium’s density and elastic properties.
- Transverse Waves: In transverse waves, the particle displacement is perpendicular to the direction of wave propagation. These waves involve oscillations that move up and down or side to side relative to the wave direction. Transverse waves primarily propagate through solids and are also known as shear waves. They do not propagate through fluids because fluids cannot support shear stress.
Performance Metrics
- Speed: Longitudinal waves generally travel faster than transverse waves in the same medium due to the different mechanisms of particle displacement and the medium’s response to compressive versus shear stress.
- Energy Transfer: Longitudinal waves can transfer energy efficiently through compressions and rarefactions, while transverse waves transfer energy through perpendicular oscillations, which can be less efficient in non-rigid media.
- Attenuation: Transverse waves tend to attenuate more quickly in non-rigid media compared to longitudinal waves, limiting their range of propagation in such environments.
Examples of Longitudinal Waves
Sound Waves: In a solid, liquid, or gas, sound waves propagate as longitudinal waves. The particles of the medium oscillate back and forth parallel to the direction of wave travel, creating compressions and rarefactions (expansions) that transmit the sound energy1.
Pressure Waves: In fluids, pressure variations can create longitudinal waves. These waves can propagate through the fluid, causing variations in pressure at different points in space.
Electromagnetic Field Waves: In the context of electromagnetic fields, longitudinal waves can refer to waves where the electric field has a component parallel to the direction of propagation. These can be described by modified electromagnetic theories that allow for longitudinal components.
Longitudinal Waves in Elastic Rods: In materials like elastic rods, longitudinal waves can be described by equations such as the Caudrey-Dodd-Gibbon (CDG) and Pochhammer-Chree (PC) equations. These waves maintain their shape over long distances, a characteristic known as soliton behavior.
Torsional Waves in Piles: In engineering, torsional waves are a type of longitudinal wave that propagates in piles under torsional load. These waves are sensitive to defects in the pile and can be used for non-destructive testing.
Longitudinal Waves in Nanoplates: At the nanoscale, the propagation of longitudinal waves in materials like nanoplates can be affected by small-scale effects, leading to unique dispersion properties.
Applications of Longitudinal Wave
Medical Applications
- Diagnostic Imaging: Ultrasound imaging utilizes high-frequency longitudinal waves to create images of internal body structures. This non-invasive technique is crucial for monitoring fetal development, diagnosing organ conditions, and guiding surgical procedures.
- Therapeutic Uses: High-intensity focused ultrasound (HIFU) employs longitudinal waves to target and destroy diseased tissue, such as tumors, without damaging surrounding healthy tissue. This method is used in treating cancers and other medical conditions.
Industrial Applications
- Non-Destructive Testing (NDT): Longitudinal waves are used in ultrasonic testing to detect flaws in materials and structures. This technique is vital for ensuring the integrity of critical components in aerospace, automotive, and construction industries.
- Material Processing: Ultrasonic welding, which uses high-frequency longitudinal waves, is employed to join materials, particularly plastics and metals, without the need for additional adhesives or fasteners. This method is widely used in electronics and automotive manufacturing.
Communication Technologies
- Acoustic Communication: Underwater communication systems rely on longitudinal sound waves to transmit information over long distances. This is essential for submarine communication and underwater exploration.
- Surface Acoustic Wave (SAW) Devices: These devices use longitudinal waves to filter and process signals in electronic circuits. SAW devices are commonly found in mobile phones, televisions, and other communication equipment.
Seismology
- Earthquake Analysis: The study of P-waves helps in determining the epicenter and magnitude of earthquakes. This information is crucial for early warning systems and understanding seismic activity.
Contactless Sensing and Monitoring
- Remote Health Monitoring: Longitudinal waves can be used for contactless heart rate monitoring and other vital signs from a distance, providing a non-invasive method for patient monitoring.
- Surveillance and Security: These waves can be employed in remote sensing and surveillance systems to detect and monitor objects or individuals without direct contact, enhancing security measures in various settings.
Acoustics and Sound Engineering
- Sound Wave Manipulation: Techniques for converting longitudinal waves to transverse waves and vice versa are used in various applications, including sound wave manipulation for better acoustics in architectural design and audio engineering.
- Noise Control: Longitudinal waves are utilized in noise control technologies to reduce unwanted sound in industrial and residential environments.
Space and Aerospace Applications
- Space Exploration: Longitudinal waves are used in various instruments and sensors for space exploration missions, aiding in the analysis of planetary surfaces and atmospheres.
- Aerospace Engineering: Ultrasonic testing with longitudinal waves ensures the structural integrity of aerospace components, which is critical for the safety and reliability of aircraft and spacecraft.
Application Cases
Product/Project | Technical Outcomes | Application Scenarios |
---|---|---|
Projection Exposure System Carl Zeiss SMT GmbH | Enhanced precision in exposing radiation-sensitive substrates using optimized pupil transmission settings. | High-precision lithography in semiconductor manufacturing. |
Therapeutic Ultrasound Device Bioness, Inc. | Improves tissue healing by controlling acoustic wave nature and alignment with targeted tissue areas. | Accelerating the healing of superficial musculo-skeletal tissue wounds. |
Focused Ultrasound System Insightec Ltd. | Utilizes both longitudinal and shear waves to focus ultrasound into the brain through the skull, enhancing spatial registration of diagnostic information. | Non-invasive brain treatments and diagnostics. |
Simulation Technique with Local Grid Refinement Fujitsu Ltd. | Improves accuracy and numerical stability of computer-implemented simulations using subgridding techniques. | Electromagnetic simulations in computational physics and engineering. |
Experimental Methods for Longitudinal Waves Southwest University | Provides new experimental methods for observing and measuring mechanical longitudinal waves. | Physical experiments and educational demonstrations in wave mechanics. |
Latest Technical Innovations in Longitudinal Wave
Innovative Materials for Longitudinal Waves
Negative Refractive Index Materials
Recent studies have focused on the transmission characteristics of ultrasonic longitudinal wave signals in negative refractive index materials. A new structure designed using COMSOL software has shown excellent acoustic longitudinal wave transmission performance. This design overcomes previous limitations and enhances sound wave focusing capabilities, which is crucial for the development of solid metamaterial structures.
Advanced Methods for Longitudinal Wave Generation and Control
Analytical Techniques for Soliton Waves
The construction of soliton waves via nonlinear Caudrey-Dodd-Gibbon (CDG) and Pochhammer-Chree (PC) equations has been examined using the Exp(−u(f))-expansion method. This method allows for the creation of solitary waves that maintain their shape after interacting with other waves, which is significant for applications requiring stable wave propagation.
Ultrasound Focusing through Skull
Systems and methods now focus ultrasound into the brain through the skull using both longitudinal and shear waves. This approach optimizes the angle of incidence, enhancing each wave’s contribution for improved diagnostic and therapeutic applications.
Modal Converter for Tissue Healing
A novel method involves using a modal converter with ultrasonic transducers positioned at various angles relative to tissue surfaces. This setup produces longitudinal waves perpendicular to the bone surface and propagates along the skin and bone surfaces. This technique enhances angiogenesis and promotes the biological healing phases of bone fractures.
FAQs
- What is the main difference between longitudinal and transverse waves?
In longitudinal waves, particles move parallel to the wave direction, while in transverse waves, particles move perpendicular to the wave direction. - Why are sound waves longitudinal?
Sound waves cause compressions and rarefactions in air molecules, leading to particle motion parallel to the wave’s propagation. - Do longitudinal waves require a medium to travel?
Yes, longitudinal waves need a medium like solids, liquids, or gases to propagate because they rely on particle vibrations. - What are compressions and rarefactions?
Compressions are regions where particles are packed closely together, while rarefactions are areas where particles are spread apart. - Can longitudinal waves travel through a vacuum?
No, longitudinal waves require a medium to propagate and cannot travel through a vacuum.
To get detailed scientific explanations of longitudinal waves, try Patsnap Eureka.
