
What is Spring Force?
Spring force is a fundamental concept in physics, describing the force exerted by a spring when it is stretched or compressed. Governed by Hooke’s Law, this force is directly proportional to the displacement from the spring’s natural length. Represented mathematically as F = -kx, where F is the spring force, k is the spring constant indicating stiffness, and x is the displacement, spring force plays a crucial role in various applications, from mechanical systems to everyday devices. This article explores the principles, calculations, and practical uses of spring force in depth.
Hooke’s Law and the Formula for Spring Force
Hooke’s Law is a key principle in physics that explains how elastic materials behave under compression or tension. It states that the force needed to stretch or compress an elastic material is proportional to the distance it is displaced. This relationship is expressed mathematically as:
F = -kx
Here:
- F represents the force exerted (in Newtons, N),
- k is the spring constant (in Newtons per meter, N/m), indicating material stiffness.
- x denotes the displacement from equilibrium (in meters, m).
This equation highlights that stiffer materials have higher k values, requiring more force for the same displacement. Hooke’s Law is widely applied in engineering, mechanics, and everyday devices.
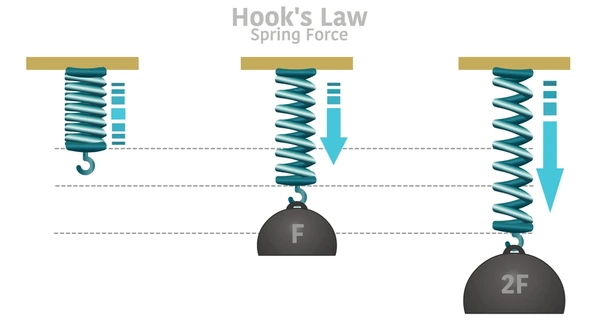
Factors Affecting Spring Force
Material Properties
- Elastic Modulus (Young’s Modulus): Higher modulus materials create stiffer springs, offering greater resistance to deformation.
- Tensile Strength: This determines the maximum load a spring can handle without breaking.
- Fatigue Strength: Springs in repeated load scenarios require materials with high fatigue resistance to avoid failure.
- Corrosion Resistance: Materials with excellent corrosion resistance are essential in harsh environments to prevent rust or chemical damage.
Geometric Factors
- Wire Diameter: Thicker wires produce stiffer springs with higher load capacity.
- Coil Diameter: Coil size impacts stiffness and the spring’s ability to bear loads.
- Number of Coils: More coils provide better load distribution but may lower spring stiffness.
- Spring Length: Longer springs allow for greater deflection but may decrease overall stiffness.
- Winding Direction: The winding direction determines compatibility and interaction when springs are used in pairs or systems.
Spring Rate (Stiffness)
The spring rate defines the force required to compress or extend the spring by a unit distance. It is determined by both material and geometric properties. A precise spring rate is critical for applications requiring accurate load-handling or deflection control.
Environmental Factors
- Temperature: High heat can reduce a material’s stiffness and strength, diminishing the spring’s force output.
- Humidity and Corrosion: Moist environments can corrode materials, impacting durability and force reliability.
- Vibration and Shock: Continuous vibration or shocks can cause fatigue, leading to potential spring failure over time.
Applications of Spring Force
Mechanical Systems
- Elevator Governors: Springs control relative positions between masses and sheaves, ensuring smooth operation. They work alongside centrifugal forces to actuate governors but face challenges like frequency interference and manufacturing tolerances.
- Suspension Systems: In vehicles, springs absorb shocks and maintain stability. Advanced neural networks now optimize their performance by adjusting amplification factors in real-time.
Medical Devices
- Administering Devices: Springs ensure reliable contact in infusion devices, maintaining proper function by securing power terminals and enabling smooth operation.
- Syringes and Injection Pens: Spring assemblies precisely regulate stopper movement, ensuring accurate and consistent medication delivery in modern injection devices.
Computational Physics and Animation
- Spring-Mass Systems: These systems simulate brittle fractures in animations, leveraging strain-based forces and mass connections for simple yet effective parallelized physical modeling.
Electromagnetic Relays
- Design Optimization: Calculating spring characteristics is critical for reliable relay performance. Deformation energy methods ensure accurate force predictions for optimal operation.
Industrial Applications
- Pouring Valves: Springs in valves prevent leaks and enhance performance. Their force characteristics directly affect reliability during beverage dispensing.
Magnetic Springs
- Innovative Design: Magnetic springs rely on calculated retracting forces instead of mechanical deformation, offering adjustable properties for specialized applications.
Energy Storage and Absorption
- Versatile Applications: Springs store potential energy when stretched or compressed, releasing it efficiently for use in diverse mechanical and engineering systems.
Application Cases
Product/Project | Technical Outcomes | Application Scenarios |
---|---|---|
Elevator Governor Otis Elevator Co. | Utilizes spring force to control the radial outward movement of masses, ensuring controlled actuation of the governor. | Elevator systems requiring precise control of movement and safety mechanisms. |
Administering Device Roche Diabetes Care, Inc. | Uses spring force to ensure electrical contact within the device, enhancing reliability and performance. | Medical devices for infusing or injecting products, ensuring consistent and reliable operation. |
Hand-held Drive-in Tool Hilti AG | Employs spring force to improve the efficiency and effectiveness of nailing and stapling tools. | Construction and manufacturing environments where portable and efficient fastening tools are required. |
Electromagnetic Relay Harbin Institute of Technology | Developed a universal calculation method for spring force characteristics, ensuring precision in engineering applications. | Design and testing of electromagnetic relays in various engineering fields. |
Pouring Valve Chongqing Technology & Business University | Analyzes spring force to ensure the valve’s structural integrity and prevent leaks. | Food and beverage industry, specifically in the design and operation of pouring machines. |
Latest Technical Innovations in Spring Force
New Materials
- High-Strength Alloys: Advanced materials like AHSS and titanium alloys have enhanced spring performance by improving strength-to-weight ratios and fatigue resistance. These alloys are ideal for demanding applications like aerospace and automotive, where durability and efficiency are essential.
- Composite Materials: Carbon fiber-reinforced polymers (CFRP) have revolutionized spring design by offering lightweight, high-strength alternatives. These composites are perfect for electric vehicles and sports equipment, where weight reduction is critical.
Manufacturing Techniques
- Additive Manufacturing: 3D printing enables the creation of springs with complex geometries and tailored performance characteristics, such as variable stiffness and optimized load distribution. This method allows for unprecedented customization and innovation.
- Surface Treatments: Techniques like shot peening and ion implantation increase surface hardness and fatigue life, making springs more resistant to wear and crack formation. These processes enhance reliability and durability in high-stress environments.
Design Innovations
- Variable Stiffness Designs: Adaptive springs with adjustable stiffness improve performance under varying loads and conditions, offering greater stability and comfort in applications like automotive suspensions.
- Micro Springs: Miniaturized springs are essential for precision in devices like sensors, actuators, and medical instruments. They deliver reliable performance in space-constrained and highly specialized systems.
To get detailed scientific explanations of spring force, try Patsnap Eureka.
