
Understanding Tensile Strength
Tensile strength is a critical property of materials, reflecting their ability to withstand tension without failure. It is defined as the maximum stress that a material can endure while being stretched or pulled before breaking. This property is essential in various industries, including construction, automotive, aerospace, and materials science, as it determines the durability and performance of materials under mechanical loads.This article delves into its structural characteristics, testing methods, and diverse applications across a wide range of industries.
Types of Tensile Strength: Yield, Ultimate, and Fracture Strength
Yield Strength
Yield strength marks the point where a material begins permanent deformation under stress. Before this, materials deform elastically, meaning they return to their original shape once the stress is removed. Beyond this point, permanent deformation occurs, impacting the material’s structural integrity. Yield strength is critical in applications like building frameworks and automotive components, where materials endure constant mechanical stress.
Example: Aluminum composites reinforced with silicon carbide (SiC) show improved yield strength as SiC content increases. For instance, a 33% rise in yield strength was observed in composites with 9% SiC reinforcement.
Ultimate Tensile Strength (UTS)
Ultimate tensile strength refers to the maximum stress a material can handle before it begins to break. It is a vital metric for comparing material performance under tension, particularly in high-stress environments like aerospace or heavy-duty machinery.
Example: Ultra-high-strength steels can achieve UTS values between 1900 and 2300 MPa. For tungsten alloys, tensile strength remains stable between 300°C and 600°C before decreasing at higher temperatures.
Fracture Strength
Fracture strength, or breaking strength, is the stress level where a material ultimately fails and fractures. This property is essential for evaluating performance in extreme conditions and ensuring safety in critical applications.
Example: Titanium alloys like Ti6Al4V demonstrate higher fracture toughness when the gap between proof and ultimate strength increases. This correlation enables improved toughness by optimizing material properties.
Measuring Tensile Strength: Methods and Techniques
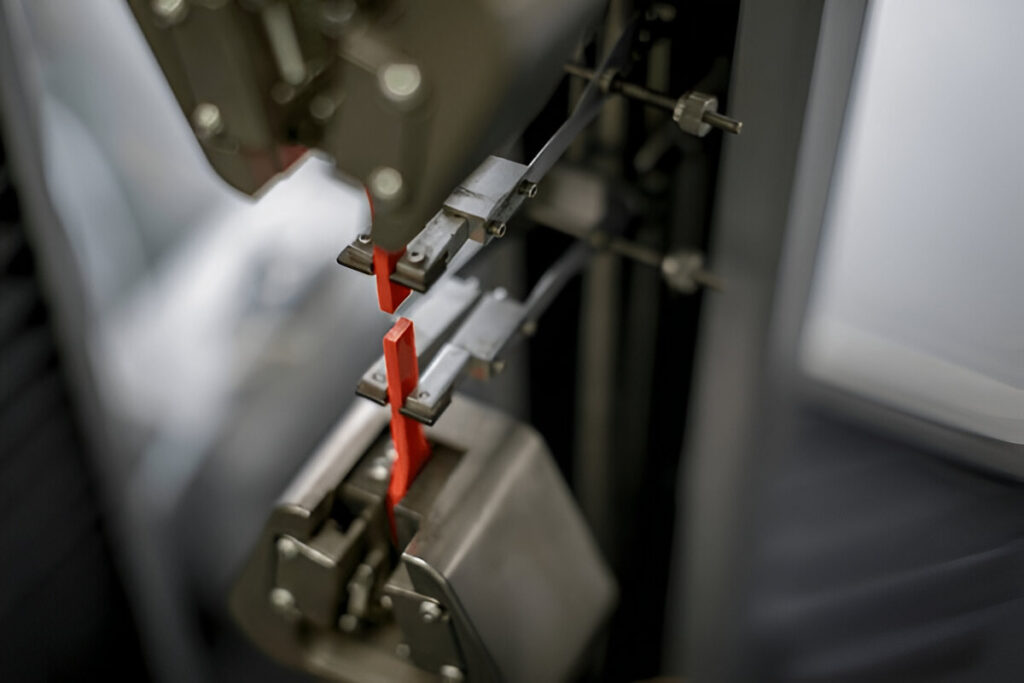
Standard Testing Methods
Universal Testing Machine (UTM):
The UTM is the most widely used device for evaluating material strength under tension. It applies a uniaxial load to the specimen until fracture occurs. Strength is calculated using the formula σ = P/A₀, where P is the maximum load and A₀ is the original cross-sectional area. This method adheres to ASTM and ISO standards, ensuring consistent and reliable results across industries.
Split Tensile Test:
This test is ideal for brittle materials like concrete. A compressive load is applied along a cylindrical specimen’s diameter until it splits. Strength is determined using the failure load and the sample’s dimensions, offering precise insights into material behavior.
Advanced Techniques for Specific Materials
Electromagnetic Heating Method:
For tight rock samples, this technique heats the material with electromagnetic energy, increasing pore-water pressure until failure. Strength is determined by analyzing pore pressure at the break point, making it essential for geomechanical studies.
Photolithography and Finite Element Analysis:
Used for low-modulus thin films like parylene-C, this method combines photolithography for sample fabrication with finite element analysis to derive material strength. It provides accurate data for delicate, high-precision materials.
Techniques for Soil and Geomaterials
Direct Tensile Strength Measurement:
This device evaluates soil and geomaterials by applying tension with grippers and plates, determining resistance to cracking. It’s crucial for assessing soil stabilization techniques and environmental durability.
Double Punch Test:
This method estimates soil sample strength by applying uniform stress distribution along a failure plane. It’s widely used for enhanced sand-bentonite mixtures treated with cement.
Specialized Methods for Brittle Materials
Roller Bending Method:
This technique measures the strength of brittle materials like glass and ceramics. It involves passing the material over rollers and applying a tensile force to induce failure, providing reliable data on material durability.
Drilling Loading Method:
For irregular, small stones, this method drills and applies load while accounting for stress concentration near the hole edge. It ensures accurate strength measurements for brittle and non-uniform materials.
Factors Affecting Tensile Strength in Materials
Intrinsic Material Properties
Composition and Microstructure:
A material’s composition and microstructure significantly influence its strength. For instance, in grey cast iron, factors like carbon content, steel scrap proportion, and graphite morphology play critical roles. Similarly, in HDPE, the tensile behavior depends heavily on how test samples are prepared and conditioned.
Alloying Elements and Reinforcements:
Adding alloying elements such as silicon and chromium boosts the strength of middle carbon steels through solid solution and work hardening. In aluminum matrix composites, reinforcements like SiC and Al₂O₃ enhance dislocation density and refine grain structures, improving overall performance.
Processing Techniques
Fabrication Parameters:
In additive manufacturing, parameters like layer thickness and build orientation significantly impact material strength. For example, FDM parts achieve greater strength with a (45°, -45°) raster angle and solid infill.
Casting Processes:
Casting integrity depends on factors like wall thickness, inclusion distribution, and porosity. These directly influence the strength and durability of the final product.
Work Hardening and Heat Treatment:
Processes like work hardening and heat treatments, including solid solution hardening, optimize material microstructure, reducing defects and enhancing strength.
Environmental Conditions
Temperature and Humidity:
Environmental factors, including temperature and humidity, can alter material behavior. For example, HDPE’s performance shifts under varying test temperatures, affecting its mechanical properties.
Corrosion and Oxidation:
Corrosive environments weaken material strength. High-strength steels counteract this with elements like molybdenum and titanium to resist delayed fractures in harsh conditions.
Application-Specific Factors
Composite Materials:
In composites, the reinforcement type and matrix bonding are critical. Natural fiber-reinforced composites show varying strengths based on fiber content and processing methods.
Polymer Composites:
Adding inorganic fillers to polymers like HDPE can enhance performance by up to 30%, demonstrating the potential of tailored material compositions for specific applications.
Tensile Strength of Common Materials: A Quick Overview
Metals
Metals are prized for their remarkable strength, making them essential for structural applications. Martensitic steels, for example, offer tensile strengths between 1700 and 2200 MPa, depending on their composition and heat treatments. Similarly, Inconel alloys like Inconel 625 and Inconel 718 exhibit strengths of about 890 MPa and 865 MPa, respectively, influenced by treatments and specimen types. Titanium alloys, particularly Ti-6Al-4V, stand out with strengths up to 1052 MPa, proving ideal for high-performance applications.
Composites
Composite materials combine distinct components to achieve outstanding mechanical properties. For instance, polyimide-based composites with 55% non-carbonaceous fillers can achieve strengths of at least 44.9 MPa. Al/SiC composites demonstrate strengths reaching 192 MPa, depending on reinforcement content and processing. Nanomaterial-reinforced composites, such as AA5083-2 wt% n-SiC nanocomposites, achieve tensile yield strengths of 224 MPa, showcasing the transformative impact of nanomaterials.
Polymers
Polymers, though generally less strong than metals or composites, are valued for their flexibility and processability. Polylactic acid (PLA) and its derivatives, such as PLLA and PDLA, offer tensile strengths from 103 MPa to 827 MPa, with typical values around 552 MPa. Biodegradable polymers, including polycaprolactone and polydioxanone, fall within a range of 103 MPa to 414 MPa, making them suitable for diverse applications.
Applications of Tensile Strength
Construction and Structural Engineering
Strength is critical for construction materials like steel and concrete. High-strength steel, with tensile values between 750 and 1250 MPa, enhances structural elements’ ductility and buckling resistance. In resistance welding and cryogen containers, steel achieves tensile strengths up to 1700 MPa. Concrete, too, benefits from innovations like rice husk ash (RHA), increasing tensile capacity by 17.65% at 15% RHA. Nanofibrous concrete with carbon nanotubes boosts axial and cleavage strength by 80% and 145%, respectively.
Automotive Industry
The automotive industry relies on strong yet lightweight materials to improve safety and performance. Steel sheets with strengths above 1320 MPa enhance fatigue resistance and bendability for critical components. Frames made of hot-rolled steel with tensile strengths over 780 MPa combine excellent ductility with superior fatigue performance.
Aerospace Industry
In aerospace, materials must withstand extreme conditions while remaining lightweight. Carbon-fiber-reinforced polymers (CFRPs) and Kevlar® excel with exceptional strength-to-weight ratios, making them ideal for reinforcement and armor applications.
Manufacturing and Industrial Applications
High-strength materials play a vital role in manufacturing. Aluminum composites reinforced with 20% SiC achieve peak tensile strength due to enhanced load transfer. In soil and geomaterials, tensile tests identify cracking risks, aiding stabilization and slope reinforcement.
Research and Development
Tensile strength drives innovations in material science. Ab initio calculations reveal the theoretical strength of metals, offering insights for advanced designs. High-tensile steels with delayed fracture resistance ensure durability for construction, pipelines, and heavy machinery.
Application Cases
Product/Project | Technical Outcomes | Application Scenarios |
---|---|---|
High-Quality Cold-Formable Ultra-Resistant Austenitic Steel Thyssen Krupp Stahl GmbH | Tensile strength up to 1,100 MPa with excellent deep-draw qualities, TRIP and TWIP properties. | Body work components, reinforcing structural components, cryogen containers, and piping. |
High-Tensile Strength Steel for Resistance Welding JFE Steel Corp. | Tensile strength of 1700 MPa or more, with nano-hardness of 5.0 GPa or less in surface layer. | Manufacturing joint structures by resistance welding, especially in automotive and heavy machinery. |
High and Ultra-High Strength Steel Tubes Monash University | Enhanced load-bearing capacities, improved strength, local buckling behavior, and ductility. | Construction practice, particularly in long hybrid compressive members. |
Porous TPU/PLA Shape Memory Polymer Blend University of Toronto | Highest tensile strength, toughness, and percentage extension with uniform pore structure. | Biomedical and clinical applications requiring a combination of tensile strength, toughness, and low density. |
Nanofibrous Concrete Belarusian National Technical University | 80% increase in axial tensile strength and 145% increase in cleavage tensile strength. | Construction applications requiring enhanced tensile properties and durability. |
To get detailed scientific explanations of tensile strength, try Patsnap Eureka.
