
Indium gallium nitride (InGaN) technology has been instrumental in the development of high-performance blue and green light-emitting diodes (LEDs). As the core material for visible-spectrum solid-state lighting, Indium gallium nitride enables compact, energy-efficient devices used in everything from smartphones and displays to automotive lighting and street lamps.
What is InGaN technology in blue and green LEDs? Eureka Technical Q&A explains that InGaN (Indium Gallium Nitride) semiconductors enable efficient light emission in the blue and green spectrum, making them essential for high-brightness displays, white LEDs, and full-spectrum lighting applications.
This article explores how Indium gallium nitride works, the science behind its tunable wavelengths, and its importance in shaping modern LED display and lighting technologies.
What Is InGaN?
InGaN (Indium Gallium Nitride) is a ternary compound semiconductor made by alloying gallium nitride (GaN) with indium nitride (InN). By varying the ratio of indium to gallium in the crystal structure, engineers can tune the material’s bandgap to emit different wavelengths of light in the visible range.
- GaN alone has a bandgap of ~3.4 eV (ultraviolet).
- InN alone has a much smaller bandgap (~0.7 eV).
- InGaN bridges this range, enabling blue (450–495 nm) and green (495–570 nm) emission.
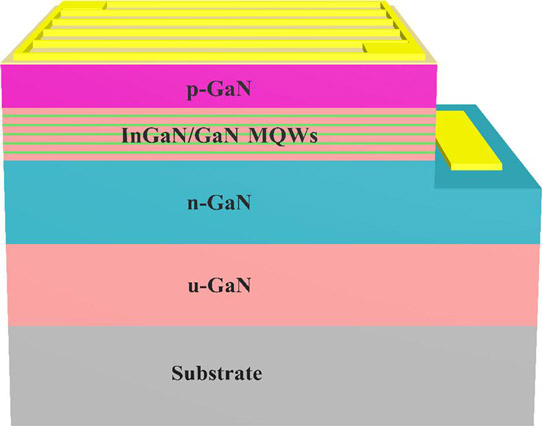
How InGaN LEDs Work
InGaN-based LEDs are semiconductor p-n junction diodes. When forward-biased, they emit light via electroluminescence — converting electrical energy into photons.
Key Layers in InGaN LEDs:
- Substrate (e.g., sapphire, SiC, or GaN)
- N-type GaN layer
- InGaN quantum wells (light-emitting region)
- P-type GaN layer
- Electrodes (for current injection)
Emission Process:
- Electrons and holes are injected into theIndium gallium nitride layer.
- They recombine in the multiple quantum wells (MQWs).
- Photons are emitted at wavelengths determined by the indium content.
More indium → lower bandgap → longer wavelength (green) Less indium → higher bandgap → shorter wavelength (blue)
Tuning Color with InGaN
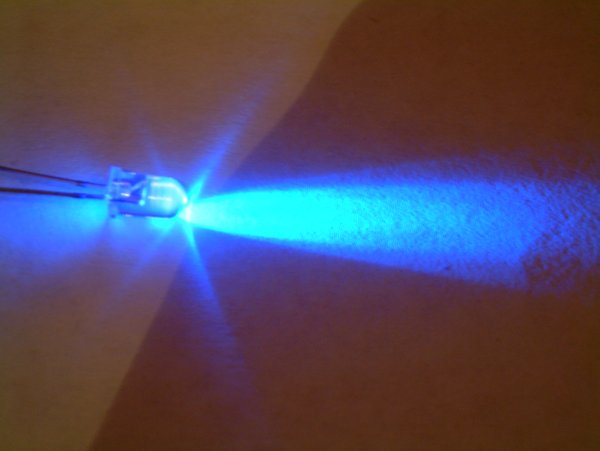
By adjusting the In/Ga ratio, Indium gallium nitride can be engineered to emit a specific color in the visible spectrum:
Emission Color | Approx. Wavelength | Indium Content in InGaN |
---|---|---|
Violet | ~420 nm | Very low |
Blue | ~450–470 nm | Low |
Cyan | ~490 nm | Moderate |
Green | ~520–550 nm | Higher |
This tunability makes Indium gallium nitride ideal for RGB display technologies and full-spectrum LED lighting.
Applications of InGaN-Based LEDs
1. Blue LEDs
- Used as the base for white light generation by adding yellow phosphor.
- Applications: general lighting, smartphone flashlights, TVs, automotive headlights.
2. Green LEDs
- Critical for true-color RGB pixel rendering in displays and signage.
- Applications: AMOLED, MicroLED, and full-color LED indicators.
3. Quantum Dot and Phosphor Excitation
- Blue InGaN LEDs serve as excitation sources for quantum dots or phosphors that convert blue light into red or green.
4. MicroLED Displays
- InGaN-based microLEDs provide high brightness, low power consumption, and ultra-small pixel sizes for next-gen displays.
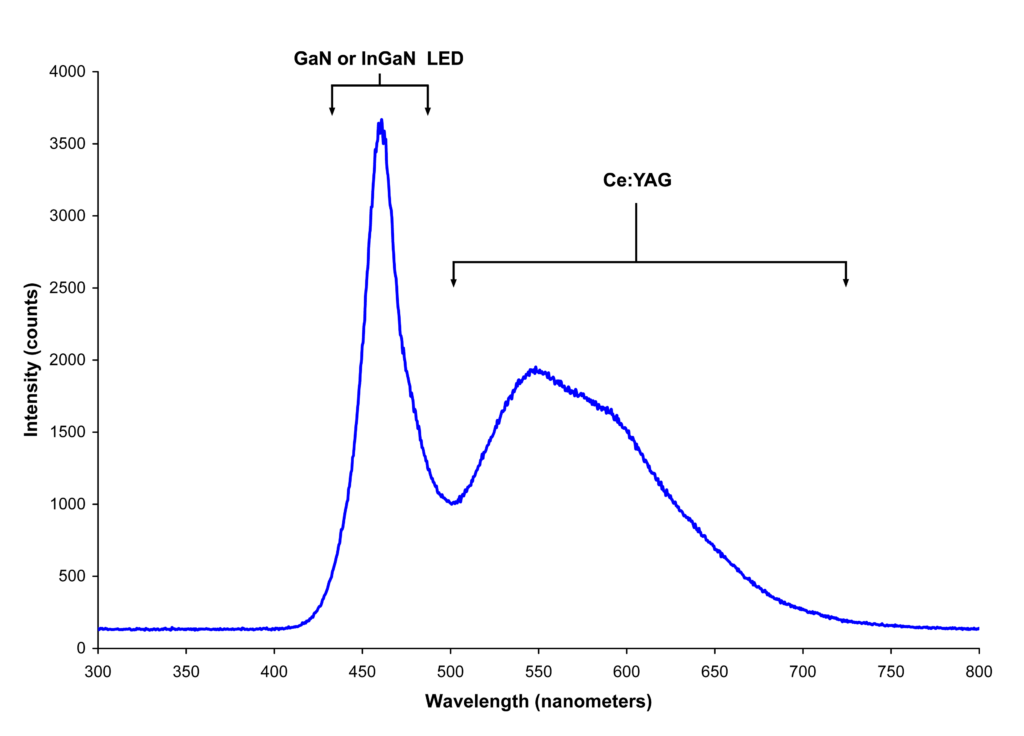
Application Cases
Product/Project | Technical Outcomes | Application Scenarios |
---|---|---|
InGaN LED EPISTAR Corp. | Enhanced light emitting efficiency and reduced series resistance through high conductivity transparent oxide layer and optimized contact layer. | High-efficiency lighting applications requiring improved performance and reduced power consumption. |
Monolithic Multi-Color LED Intel Corp. | Improved efficiency and reduced manufacturing costs through enhanced InGaN material quality using Hydrazine for lower temperature layer formation. | Applications requiring high-quality, cost-effective multi-color LED displays. |
Near UV LED Seoul Viosys Co., Ltd. | Improved light output and crystallinity through integration of superlattice layer and electron implantation layer. | Applications requiring high-performance near-ultraviolet light emission, such as sterilization or curing processes. |
Semipolar GaN LED University of California, Santa Barbara | Achieved luminous efficacy greater than 150 lm/W and external quantum efficiencies higher than 60% at low current densities. | Energy-efficient solid-state lighting and high-performance display technologies. |
Freestanding Semipolar InGaN LED Tyndall National Institute | Bandwidth in excess of 150 MHz at 20 mA, enabling 300 Mbps back-to-back transmission. | Visible light communication (VLC) applications requiring high-speed data transmission. |
Benefits of InGaN Technology
- Tunable Bandgap: One of the most significant benefits of Indium gallium nitride is its ability to have a tunable bandgap by adjusting the indium composition. This allows it to cover a wide range of wavelengths, from the visible to the ultraviolet spectrum, making it suitable for various optoelectronic applications such as LEDs, lasers, and photodetectors.
- High Electron Mobility and Thermal Stability: Indium gallium nitride exhibits high electron mobility and good thermal stability, which are essential for power electronics and high-frequency applications. These properties contribute to the efficient operation of devices under harsh conditions.
- Efficiency and Performance Enhancement: InGaN-based devices often show improved efficiency and performance compared to traditional semiconductor materials. For instance, InGaN LEDs with multilayer barriers demonstrate higher light output power, lower current leakage, and reduced efficiency droop.
Feature | Benefit |
---|---|
Bandgap Tunability | Enables multiple colors from one material system |
High Brightness | Efficient radiative recombination |
Long Lifespan | Reliable solid-state construction |
Low Power Consumption | Energy-efficient light generation |
Compact Size | Suitable for small-scale displays |
Challenges in InGaN LED Development
While InGaN LEDs are essential, they come with certain material and fabrication challenges:
1. Lattice Mismatch
- The difference in lattice constants between GaN and InN leads to strain and defects in InGaN layers, especially at high indium content (used for green emission).
2. Efficiency Droop
- At high current densities, efficiency drops due to non-radiative recombination and carrier leakage.
3. Thermal Management
- Heat generation affects performance and longevity; advanced packaging and heat sinks are required.
4. Green Gap
- Achieving high-brightness true green (around 530 nm) is difficult due to low radiative efficiency — a phenomenon known as the “green gap.”
Comparison: InGaN vs AlGaInP LEDs
Parameter | InGaN LEDs | AlGaInP LEDs |
---|---|---|
Emission Range | Blue to green | Red to yellow |
Brightness (Green) | Lower (green gap) | Brighter in red/orange |
Lifetime | Longer | Shorter (thermal issues) |
Efficiency at High Current | Better for blue | Poorer for high-current |
Common Use | Displays, lighting | Traffic lights, indicators |
Future of InGaN LEDs
Innovations in substrate engineering, strain management, and nanostructures are improving InGaN performance:
- GaN-on-GaN substrates: reduce defects, improve thermal conductivity.
- Nanowire LEDs: offer better strain relaxation for high-indium green emission.
- Hybrid perovskite-InGaN systems: potential for broader spectrum emission.
- MicroLED and AR/VR display advancements: rely heavily on high-resolution InGaN arrays.
With these advancements, InGaN will remain central to next-gen lighting, wearable displays, and optical communication technologies.
Conclusion
InGaN technology is the foundation of modern blue and green LED innovation. By fine-tuning its indium content, engineers can produce efficient, tunable visible light essential for display systems, lighting solutions, and photonic devices. Despite challenges like the green gap and efficiency droop, InGaN continues to evolve through material science and nanotechnology — positioning it as a cornerstone of the LED revolution.
FAQs
InGaN stands for Indium Gallium Nitride, a compound semiconductor used in LEDs.
It enables blue light emission by providing a suitable bandgap (~2.7 eV) when doped correctly.
InGaN is an alloy that includes indium, which allows bandgap tuning. GaN alone is used primarily for UV or violet LEDs.
Due to lattice mismatch and strain from high indium content, resulting in more defects and non-radiative losses — the “green gap.”
Yes, most white LEDs use blue InGaN LEDs coated with yellow phosphor to produce broad-spectrum white light.
To get detailed scientific explanations of InGaN, try Patsnap Eureka.
