
Phosphorescence is a type of photoluminescence where certain materials absorb energy and then re-emit it as visible light over an extended period, even after the energy source is removed. Unlike fluorescence, which stops immediately when the excitation source disappears, phosphorescent materials glow in the dark for seconds, minutes, or even hours. This phenomenon has a wide range of applications in safety signs, glow-in-the-dark products, display technologies, and scientific imaging. This article explores how phosphorescence works, the types of materials involved, industrial uses, and key comparisons with related optical processes.
What is phosphorescence? Eureka Technical Q&A explains this light-emitting phenomenon, where certain materials absorb energy and re-emit it slowly over time, commonly used in glow-in-the-dark products, safety signs, and scientific applications.
How Phosphorescence Works
Phosphorescence involves a delay in the re-emission of absorbed light energy due to the quantum mechanics of excited electron states. Here’s a breakdown of the process:
- Excitation – When exposed to UV or visible light, electrons in a phosphorescent material absorb energy and move from a ground state to an excited singlet state.
- Intersystem Crossing – Instead of returning directly to the ground state (as in fluorescence), some electrons undergo a spin transition into a triplet state, a forbidden energy state that slows down relaxation.
- Delayed Emission – Electrons eventually return to the ground state, releasing energy in the form of visible light over a longer timescale.
This process makes phosphorescence time-dependent, with light emission occurring well after the excitation source is removed.
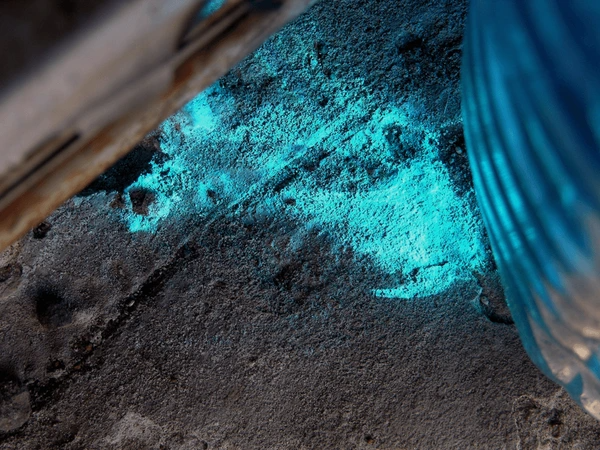
The Main Difference Between Fluorescence and Phosphorescence
While both are forms of luminescence, the key distinction lies in their time scales and electronic transitions:
- Fluorescence occurs when an electron absorbs light and jumps to an excited singlet state, then quickly returns to the ground state, emitting light within nanoseconds.
- Phosphorescence involves an additional transition to a triplet state before returning to the ground state, which is a “forbidden” transition that occurs much more slowly.
This is why phosphorescent materials continue glowing after the light source is turned off, while fluorescent ones do not.
Characteristic | Fluorescence | Phosphorescence |
---|---|---|
Emission Duration | Immediate (ns to µs) | Delayed (ms to hours) |
Light Afterglow | No | Yes |
Electron Transition | Singlet to singlet | Triplet to singlet |
Energy Loss Speed | Fast | Slow |
Common Use | Dye markers, LEDs | Glow-in-the-dark items |
Phosphorescent vs. Fluorescent vs. Chemiluminescent
Property | Phosphorescence | Fluorescence | Chemiluminescence |
---|---|---|---|
Light Duration | Long (seconds to hours) | Short (nanoseconds) | Varies, often long |
Energy Source | Light (usually UV) | Light | Chemical reaction |
Afterglow | Yes | No | Often yes |
Typical Materials | Zinc sulfide, strontium aluminate | Fluorescein, rhodamine | Luminol, oxalate esters |
Materials That Exhibit Phosphorescence
Several materials are capable of producing persistent afterglow. Common classes include:
Inorganic Phosphors
- Strontium Aluminate: Modern glow-in-the-dark pigments; brighter and longer-lasting than older materials.
- Zinc Sulfide: Traditional phosphorescent pigment, typically doped with copper.
- Rare Earth-Doped Oxides: Used in high-performance displays and safety materials.
Organic Phosphorescent Compounds
- Aromatic Carbonyls and Halides: Certain polymers and dyes can show room-temperature phosphorescence (RTP).
- Heavy Atom Substitution: Introducing atoms like iodine or bromine into molecules enhances intersystem crossing and promotes phosphorescence.
Industrial and Scientific Applications
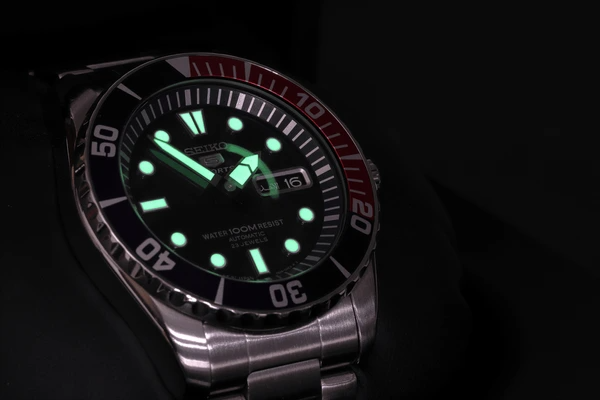
Display Technology:
- Vacuum Fluorescent Displays (VFD), Field Emission Displays (FED), and Plasma Display Panels (PDP): Phosphors are used in these displays to convert electrical energy into visible light. They are excited by electron beams or electrical current to produce images.
- Light-Emitting Diodes (LEDs): Manufacturers use phosphors in LEDs to convert shorter wavelength light (blue or ultraviolet) into longer wavelengths like white or colored light. This is crucial for white LED illumination, which has advantages like energy efficiency and longer lifespan compared to traditional lighting.
Illumination:
- White LEDs: Phosphors are key components in white LEDs, which are used in a variety of lighting applications due to their efficiency and durability. They are excited by blue or ultraviolet light emitted by a semiconductor device.
- Fluorescent Lamps: Phosphors are used in fluorescent lamps to convert electrical energy into visible light. They are excited by ultraviolet light emitted by a fluorescent coating.
Scientific Research:
- Luminescence Thermometry and Manometry: Phosphors can be used to measure temperature and pressure with high sensitivity. This is useful in various research areas, including bioimaging, catalysis, and geological processes.
- Bioimaging: Phosphorescent materials can be used as luminescent probes for real-time bioimaging. They are useful in minimizing background autofluorescence and providing clear images.
Industrial Applications:
- Emergency Signage: Designers use phosphorescent pigments in exit signs and safety markings to ensure visibility in low-light conditions.
- Coatings and Inks: Manufacturers use phosphorescent materials in coatings and inks for watch dials and decorative elements to provide long-lasting visibility.
Optoelectronic Devices:
Organic Light-Emitting Diodes (OLEDs): Engineers use phosphorescent materials in OLEDs to improve efficiency by harvesting both singlet and triplet excitons. This leads to higher efficiency and brighter displays.
Security and Anti-Counterfeiting:
Phosphorescent materials can be used in security inks and features that are visible under certain lighting conditions, making them difficult to counterfeit.
Application Cases
Product/Project | Technical Outcomes | Application Scenarios |
---|---|---|
Ce-doped β-Si3N4 Phosphor National Institute for Materials Science IAI | Improved luminance and durability for lighting and display applications | Luminescent and image display devices |
Nitride Phosphor Nichia Corp. | Enhanced red component and brightness in white light emitting devices | White LEDs with improved color temperature and rendering |
Magnetoplumbite-type Phosphor Maxell Ltd. | Improved brightness and reduced phosphorescence | Gas discharge and display devices |
Nanophosphor Composite Scintillators Los Alamos National Security LLC | Enhanced brightness and detection efficiency for radiation detection | Cost-effective radiation detection applications |
Luminescent Horological Component The Swatch Group Research & Development Ltd. | Durable and visibly effective luminescent component with enhanced performance | Horological applications with improved design flexibility |
Challenges and Limitations
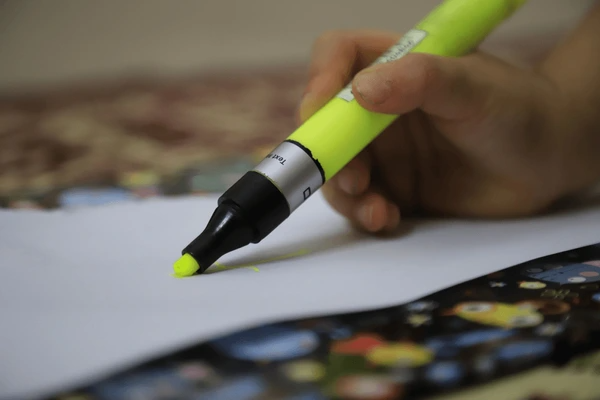
- Material Stability and Performance Trade-offs: Ceramic phosphors, for instance, offer stability but suffer from performance limitations. They are not soluble in the matrix that encapsulates light-emitting diode (LED) dies, which can affect their efficiency and the ability to mimic natural light curves.
- Solubility Issues: Organic fluorescers generally do not face solubility issues, unlike ceramic phosphors. This solubility problem can hinder the uniform distribution of phosphors within a matrix, affecting the overall performance of the device.
- Spectral Distribution Challenges: Achieving the desired spectral distribution is challenging for ceramic phosphors. They struggle to replicate the photopic light curve, which is essential for applications requiring natural light imitation.
- Environmental and Resource Concerns: The reliance on rare earth elements for phosphors poses significant environmental and geopolitical risks. There are concerns about resource scarcity, recycling challenges, and the environmental impact of mining and processing these materials.
- Color Quality Issues: Ensuring consistent color quality across different batches and applications is another challenge. Variations in the composition of phosphors can lead to differences in color output, affecting the uniformity of displays and lighting solutions.
- Scattering and Degradation: In LED applications, scattering within the phosphor layer can occur, leading to non-uniform light distribution. Additionally, some materials like titanium dioxide, used as scattering agents, can be photo-active and cause molecular degradation.
- Technological Evolution: The rapid evolution of cyber-physical systems and technologies challenges traditional summarization and understanding of phosphorescent materials, making it difficult to keep up with the latest advancements and applications.
FAQs
What is the main difference between fluorescence and phosphorescence?
Fluorescence emits light almost immediately after excitation and stops when the source is removed. Phosphorescence continues emitting light for seconds to hours due to slower electronic transitions.
Why do glow-in-the-dark items need to be “charged”?
They need to absorb energy from an external light source to excite electrons. Once the energy is stored, it can be released gradually as visible light.
What material glows the longest?
Strontium aluminate doped with rare-earth metals like europium and dysprosium currently holds the record for the brightest and longest-lasting glow-in-the-dark effects.
Can phosphorescence occur in nature?
It’s rare but possible. Some minerals exhibit natural phosphorescence, and certain biological systems can mimic delayed luminescence, although most natural glowing is bioluminescent or fluorescent.
Is phosphorescence harmful?
The phenomenon itself is not harmful. However, care should be taken regarding the chemical composition of phosphorescent materials, especially if used in consumer products.
Conclusion
Phosphorescence is a fascinating and useful luminescent process with diverse real-world applications—from life-saving safety signage to cutting-edge OLED technologies. While it has its limitations, ongoing research into new materials, particularly room-temperature organic phosphors, continues to expand its potential. Understanding how phosphorescence works allows designers, scientists, and engineers to harness its glow for innovation and safety across many sectors.
To get detailed scientific explanations of phosphorescence, try Patsnap Eureka.
