
Solid-State Lithium Dealloying Background and Objectives
Solid-state lithium dealloying has emerged as a critical area of research in the field of energy storage and materials science. This technology holds immense potential for revolutionizing battery performance and safety, particularly in the context of solid-state batteries. The process of lithium dealloying, which involves the extraction of lithium from alloys or intermetallic compounds, plays a crucial role in the development of high-performance electrode materials. Understanding the influence of pressure on this process is paramount to optimizing battery efficiency and longevity.
Diving into the effects of pressure on lithium dealloying in solid-state systems? Eureka Technical Q&A connects you with materials science and electrochemistry experts who can help interpret data, explain phase behavior, and guide you through complex research questions—making high-level battery science more accessible and actionable.
The evolution of lithium dealloying technology can be traced back to the early studies on lithium-based alloys for battery applications. Initially, the focus was primarily on liquid electrolyte systems, but the shift towards solid-state batteries has brought new challenges and opportunities. The pressure effects on lithium dealloying in solid-state systems have gained significant attention due to their potential to enhance lithium diffusion kinetics and structural stability.
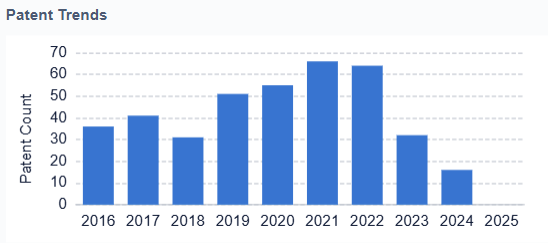
The primary objectives of this research are multifaceted. Firstly, it aims to elucidate the fundamental mechanisms by which pressure influences the lithium dealloying process in solid-state environments. This includes investigating how pressure affects the thermodynamics and kinetics of lithium extraction, as well as its impact on the structural integrity of the host materials. Secondly, the research seeks to identify optimal pressure conditions that can maximize the efficiency of lithium dealloying while minimizing degradation of the electrode materials.
Another crucial goal is to explore the potential for pressure-induced phase transformations during the dealloying process, which could lead to the discovery of novel materials with enhanced electrochemical properties. Additionally, this research aims to develop predictive models that can accurately describe the pressure-dependent behavior of lithium dealloying, enabling more efficient design of solid-state battery systems.
The technological trajectory in this field is expected to lead to significant improvements in solid-state battery performance, including higher energy density, faster charging rates, and extended cycle life. By understanding and harnessing the pressure effects on lithium dealloying, researchers anticipate breakthroughs in overcoming current limitations of solid-state batteries, such as interfacial resistance and slow lithium ion transport.
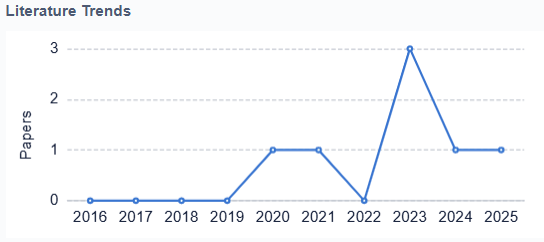
As the demand for high-performance energy storage solutions continues to grow across various sectors, including electric vehicles and renewable energy systems, the insights gained from this research will be instrumental in shaping the future of battery technology. The ultimate aim is to contribute to the development of next-generation solid-state batteries that can meet the increasing energy demands of modern society while ensuring safety and sustainability.
Market Demand for Advanced Solid-State Batteries
The solid-state battery market is experiencing a surge in demand, driven by the growing need for safer, more energy-dense, and longer-lasting energy storage solutions. This demand is particularly pronounced in the electric vehicle (EV) sector, where advanced solid-state batteries are seen as a potential game-changer. The global market for solid-state batteries is projected to grow at a CAGR of over 30% in the coming years, with estimates suggesting it could reach $6-8 billion by 2030.
The automotive industry is the primary driver of this demand, as major car manufacturers are investing heavily in solid-state battery technology to overcome the limitations of current lithium-ion batteries. These advanced batteries promise higher energy density, faster charging times, improved safety, and longer lifespan, all of which are critical factors for widespread EV adoption. Additionally, the consumer electronics sector is showing increasing interest in solid-state batteries for smartphones, laptops, and wearable devices, further expanding the market potential.
The pressure-induced lithium dealloying process in solid-state batteries is of particular interest as it directly impacts the performance and longevity of these advanced energy storage systems. Understanding and controlling this process could lead to significant improvements in battery efficiency and lifespan, addressing key market demands for longer-range EVs and more durable electronic devices.
However, the market demand for advanced solid-state batteries extends beyond just performance improvements. There is a growing emphasis on sustainability and environmental concerns, driving the need for batteries with reduced environmental impact throughout their lifecycle. This includes the use of more abundant and less toxic materials, as well as improved recyclability.
The aerospace and defense sectors are also emerging as potential high-value markets for solid-state batteries, particularly for applications requiring high energy density and safety in extreme conditions. The increasing electrification of aircraft systems and the development of electric vertical takeoff and landing (eVTOL) vehicles are creating new opportunities for advanced battery technologies.
As the technology matures and production scales up, cost reduction will be a critical factor in meeting market demand. Currently, the high cost of solid-state batteries is a significant barrier to widespread adoption. Research into pressure effects on lithium dealloying could potentially lead to more efficient manufacturing processes, helping to address this challenge.
In conclusion, the market demand for advanced solid-state batteries is robust and multifaceted, driven by the automotive industry but with significant potential across multiple sectors. The research on pressure-induced lithium dealloying is well-aligned with this demand, as it addresses key performance and manufacturing challenges that need to be overcome to meet the growing market expectations for next-generation energy storage solutions.
Pressure Effects on Lithium Dealloying: Current Understanding
Researchers are now focusing heavily on how pressure affects lithium dealloying in solid-state systems. This topic has become crucial for improving energy storage and material performance in lithium-based technologies.
Recent studies combine experimental data with theoretical models to explain pressure’s role in lithium dealloying. Pressure significantly impacts both the kinetics and thermodynamics of this process, especially in solid-state battery materials.
One major discovery is that moderate pressure often improves lithium extraction. It lowers the activation energy, allowing lithium ions to move more freely. As a result, the dealloying rate increases, enhancing battery efficiency.
However, applying too much pressure can cause problems. Excessive force may change a material’s structure or trigger unwanted phase transformations. These changes can slow or block the dealloying process.
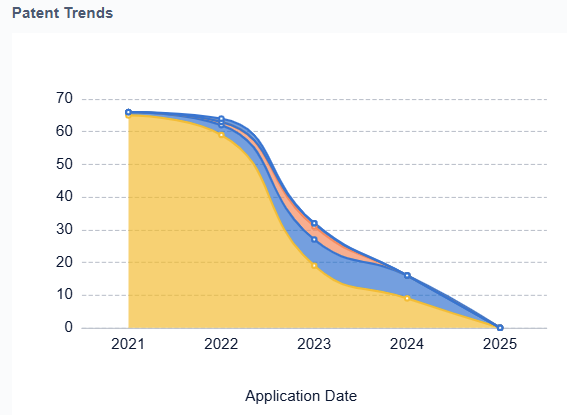
Pressure also affects how lithium ions move within the solid matrix. It can modify diffusion pathways, sometimes helping and sometimes hindering lithium movement. These effects depend on the material and the applied pressure level.
In addition, pressure influences the interface between the lithium phase and the surrounding matrix. This interface is critical for controlling the dealloying mechanism and overall battery performance.
Some researchers have observed that pressure can alter the way dealloying fronts develop. It may lead to more uniform or more localized lithium extraction depending on the system. These changes influence how the material behaves during charge and discharge cycles.
Pressure also plays a role in stabilizing the solid electrolyte interphase (SEI) layer. This layer is vital for battery lifespan and cycling stability, making pressure control a key design factor.
The interaction between pressure, temperature, and electrochemical potential adds further complexity. These variables work together to influence lithium’s chemical potential and its tendency to dealloy.
This understanding has inspired new pressure-modulated dealloying techniques. Engineers use these strategies to improve lithium extraction efficiency and extend cycle life in solid-state systems.
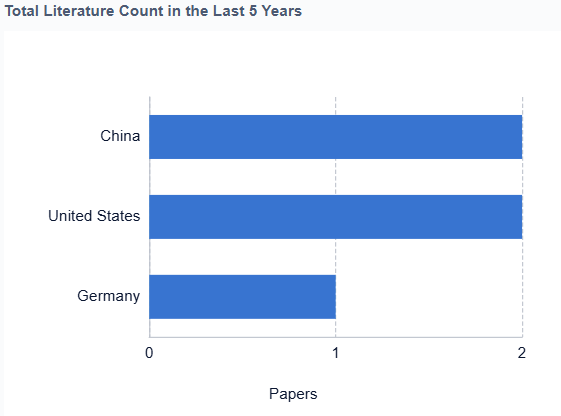
Even with these advances, knowledge gaps remain. Scientists still struggle to predict how pressure affects lithium behavior at the nanoscale or in mixed-material systems.
More targeted research is needed to control pressure-driven effects with precision. This includes developing models that better reflect real-world materials and battery designs.
Ultimately, mastering pressure’s role in lithium dealloying could lead to more efficient, durable, and reliable solid-state batteries for future energy storage solutions.
Evolution of Solid-State Battery Technologies
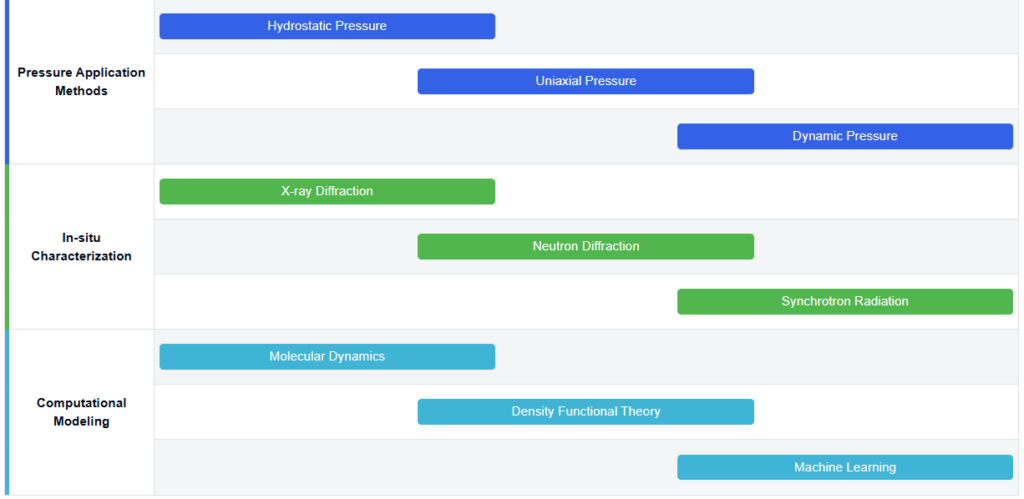
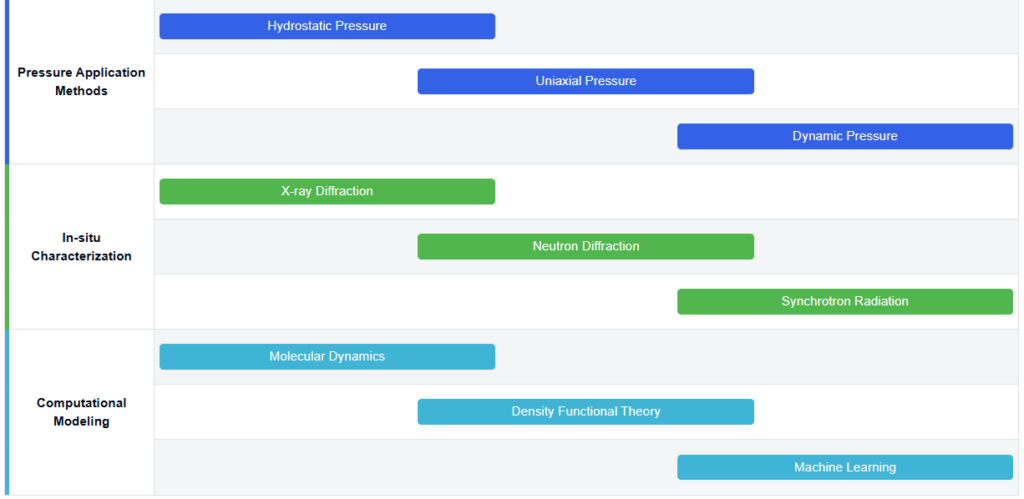
Key Players in Solid-State Battery Research
The research on pressure influence on lithium dealloying in solid-state batteries is in an early developmental stage, with significant potential for market growth. The technology is still maturing, as evidenced by the diverse range of players involved, including major automotive manufacturers like Toyota, BMW, and Hyundai, as well as specialized battery companies such as QuantumScape and LG Energy Solution. Academic institutions like Xiamen University and the University of Maryland are also contributing to advancements in this field. The market size is expected to expand rapidly as solid-state batteries offer promising solutions for energy storage challenges. However, the technology’s commercial viability is still being established, with companies like Samsung SDI and Toyota leading the way in research and development efforts.
LG Energy Solution Ltd.
Technical Solution
LG Energy Solution has developed a solid-state battery technology that utilizes a sulfide-based solid electrolyte. Their approach focuses on improving the interface between the solid electrolyte and electrodes to enhance lithium ion conductivity. They have implemented a novel coating technique on the cathode particles to create a stable interface layer, which helps to prevent unwanted side reactions and improves the overall battery performance. Additionally, they are exploring the use of composite electrolytes that combine the benefits of sulfide and oxide materials to achieve better mechanical properties and ionic conductivity.
Strengths: High energy density, improved safety, and potential for fast charging.
Weaknesses: Challenges in mass production and cost-effectiveness.
QuantumScape Corp.
Technical Solution
QuantumScape has developed a proprietary solid-state lithium-metal battery technology that uses a ceramic separator. Their approach eliminates the need for a conventional separator and liquid electrolyte, replacing them with a solid-state ceramic material. This ceramic separator allows lithium ions to pass through during charge and discharge while preventing the formation of dendrites. QuantumScape’s technology also incorporates a lithium-metal anode, which is formed in situ during the first charge cycle. This design aims to significantly increase energy density while maintaining safety and long cycle life. The company has also focused on developing manufacturing processes that can be scaled up for mass production.
Strengths: High energy density, fast charging capability, and potential for long cycle life.
Weaknesses: Challenges in scaling up production and ensuring consistent performance across large-format cells.
Samsung SDI Co., Ltd.
Technical Solution
Samsung SDI has been working on solid-state battery technology using a combination of sulfide and oxide-based solid electrolytes. Their approach focuses on developing a hybrid electrolyte system that combines the high ionic conductivity of sulfide materials with the stability of oxide materials. Samsung SDI has also been exploring the use of silicon-based anodes in conjunction with their solid electrolyte to further increase energy density. They have developed proprietary coating technologies for both the cathode and anode materials to improve the interface between the electrodes and the solid electrolyte, which is crucial for efficient lithium ion transport and overall battery performance.
Strengths: Potential for high energy density and improved safety.
Weaknesses: Challenges in achieving consistent performance and scalability.
Toyota Motor Corp.
Technical Solution
Toyota has been a pioneer in solid-state battery research, with a focus on developing all-solid-state batteries (ASSBs) for automotive applications. Their approach utilizes a sulfide-based solid electrolyte, which offers high ionic conductivity. Toyota’s technology incorporates a lithium metal anode to achieve high energy density. They have developed proprietary manufacturing techniques to address the challenges of producing large-format cells, including methods to reduce interfacial resistance between the solid electrolyte and electrodes. Toyota has also been working on improving the mechanical properties of their solid electrolyte to enhance durability and safety under various operating conditions.
Strengths: Extensive research experience, potential for high energy density, and focus on automotive applications.
Weaknesses: Challenges in scaling up production and reducing costs for mass-market adoption.
Solid Energies, Inc.
Technical Solution
Solid Energies has developed a unique approach to solid-state batteries that combines a lithium metal anode with a hybrid solid-liquid electrolyte. Their technology, known as the “quasi-solid-state” battery, uses a porous solid electrolyte structure infused with a small amount of liquid electrolyte. This design aims to combine the high energy density of lithium metal anodes with improved ionic conductivity and interfacial contact. The company has focused on developing manufacturing processes that can be integrated into existing lithium-ion battery production lines, potentially reducing the barriers to commercialization. Solid Energies has also been working on advanced cathode materials to further increase energy density and improve overall battery performance.
Strengths: Potential for high energy density, compatibility with existing manufacturing processes. Weaknesses: Challenges in long-term stability and safety due to the presence of liquid electrolyte.
Existing Methods for Studying Pressure Effects
Lithium dealloying pressure control methods
Various techniques are employed to control the pressure during lithium dealloying processes. These methods aim to optimize the extraction of lithium from alloys while maintaining structural integrity. Pressure control can involve adjusting gas pressure, using specific solvents, or applying mechanical forces to facilitate efficient lithium removal.
- Pressure control in lithium dealloying processes
Various methods are employed to control pressure during lithium dealloying processes. These techniques aim to optimize the extraction of lithium from alloys or compounds while maintaining the integrity of the material structure. Pressure control can influence the efficiency and yield of the dealloying process, as well as the properties of the resulting materials. - Electrochemical dealloying techniques for lithium extraction
Electrochemical methods are utilized for lithium dealloying, involving the application of electrical potential to facilitate the removal of lithium from alloys or compounds. These techniques often incorporate pressure control mechanisms to enhance the efficiency of lithium extraction and manage the structural changes in the material during the process. - Pressure-assisted lithium recovery systems
Specialized systems are developed to recover lithium using pressure-assisted techniques. These systems may involve the use of controlled pressure environments or pressure gradients to facilitate the extraction of lithium from various sources, including alloys, ores, or recycled materials. The pressure control in these systems plays a crucial role in optimizing the recovery process. - Pressure regulation in lithium processing equipment
Equipment used in lithium processing, including dealloying operations, often incorporates pressure regulation mechanisms. These mechanisms are designed to maintain optimal pressure conditions throughout the process, ensuring consistent and efficient lithium extraction while minimizing potential damage to the equipment or processed materials.
Electrochemical dealloying of lithium alloys
Electrochemical techniques are utilized for lithium dealloying, involving the application of electric current to extract lithium from alloy materials. This process can be controlled by adjusting parameters such as current density, electrolyte composition, and electrode potential to achieve optimal lithium removal while minimizing structural damage to the host material.Expand
Pressure-assisted lithium extraction from batteries
Pressure-assisted methods are developed for extracting lithium from spent batteries or lithium-containing materials. These techniques involve applying controlled pressure to enhance the efficiency of lithium removal, potentially combined with other processes such as chemical leaching or thermal treatment to improve overall recovery rates.Expand
Lithium dealloying in advanced energy storage systems
Research focuses on lithium dealloying processes in advanced energy storage systems, such as next-generation batteries and capacitors. These studies aim to understand and control the pressure effects during charge-discharge cycles, which can impact the performance and longevity of energy storage devices.Expand
Pressure effects on lithium alloy microstructure
Investigations are conducted on how pressure during dealloying affects the microstructure of lithium alloys. This research aims to understand the relationship between applied pressure, dealloying kinetics, and the resulting material properties, which is crucial for developing more efficient and durable lithium-based materials for various applications.
Core Innovations in Lithium Dealloying Mechanisms
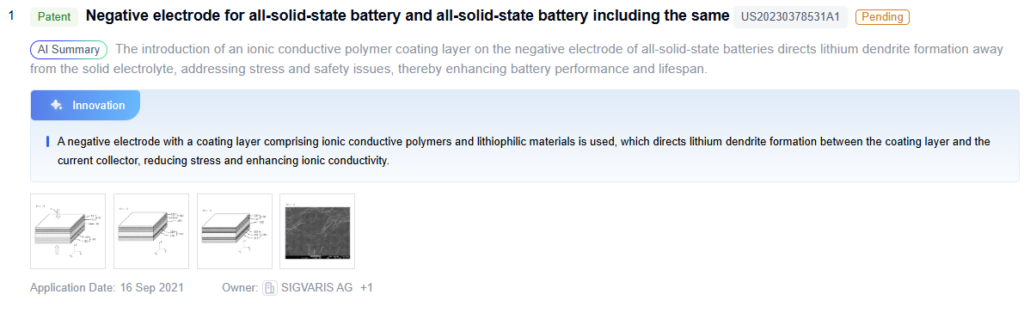
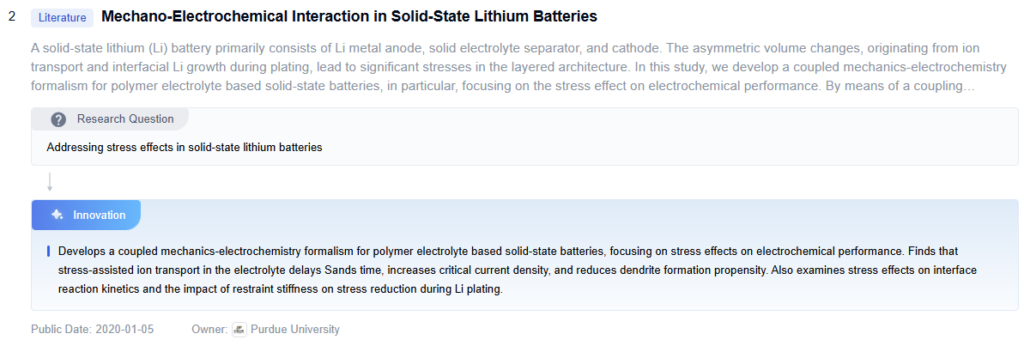
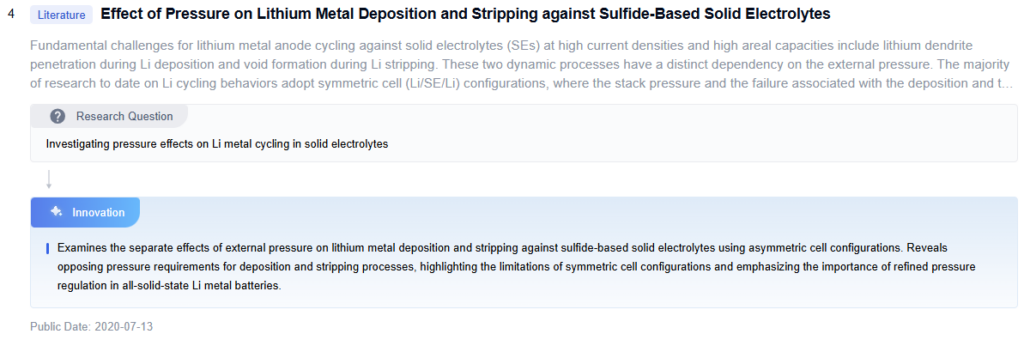
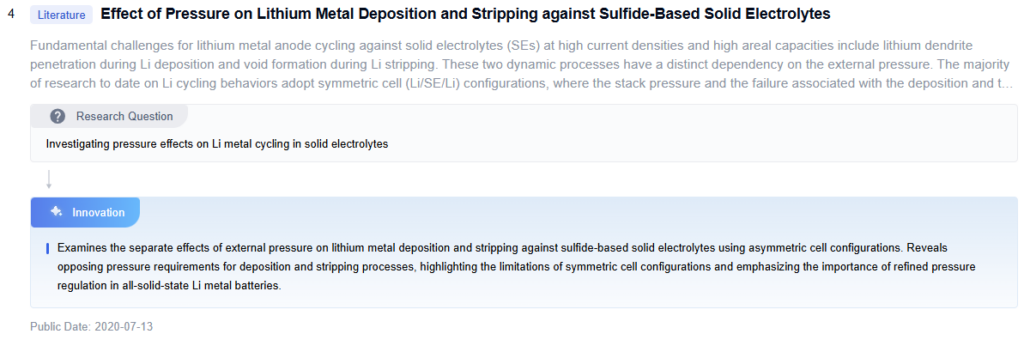
Future Directions in Pressure-Controlled Dealloying
High-Pressure Dealloying Optimization
High-pressure dealloying optimization is emerging as a powerful method to improve lithium extraction in solid-state batteries. By applying controlled pressure, researchers can enhance dealloying efficiency and achieve more uniform material performance.
This technique uses specialized pressure chambers to maintain consistent pressure during the dealloying process. These systems regulate pressure levels precisely, usually ranging from a few to several hundred atmospheres. The added pressure boosts lithium ion mobility, which increases the reaction rate and promotes even dealloying across the material.
One major advantage of this approach is its customizable pressure profile. Researchers can adjust the pressure at different stages to target specific phases or regions of the material. This control reduces issues like incomplete dealloying or uneven lithium extraction—both common problems in traditional methods.
Moreover, high-pressure dealloying works well with other optimization techniques. Combining pressure control with temperature regulation or electrochemical methods creates a multi-parameter system. This synergy allows scientists to fine-tune materials for maximum performance and stability.
To implement this method, engineers must build advanced high-pressure equipment. They need durable pressure chambers, accurate control systems, and tools for real-time monitoring under pressure. In-situ characterization methods play a key role by helping researchers observe material behavior during the process.
Additionally, computational modeling supports this technique. Simulations help predict how materials respond to varying pressures, guiding researchers in designing better experiments and materials.
As research advances, high-pressure dealloying optimization could revolutionize solid-state battery development. This approach may lead to faster lithium extraction, improved battery performance, and longer cycle life.
Strengths of this method include greater control over dealloying, improved lithium efficiency, and better uniformity. It also integrates well with other enhancement strategies.
Challenges include the need for specialized equipment, added safety concerns, and increased manufacturing complexity. Despite these hurdles, the potential benefits make high-pressure dealloying a valuable tool for next-generation energy storage.
Safety and Performance Implications
Understanding how pressure affects lithium dealloying is essential for designing safe and high-performance solid-state batteries. Pressure directly influences mechanical stability, electrochemical behavior, and long-term reliability during battery operation.
Mechanical Stress and Safety Risks
During lithium dealloying, applied pressure can introduce mechanical stress and strain in the battery’s solid components. If not properly managed, this stress may cause cracks in the solid electrolyte, leading to structural failure. These defects increase the risk of short circuits, thermal events, or other serious battery malfunctions.
Performance Benefits of Controlled Pressure
On the positive side, applying controlled pressure can accelerate lithium extraction by improving reaction kinetics. This results in faster charging and higher power output. Pressure also improves interfacial contact between electrodes and electrolytes, which enhances ion transport and overall battery performance.
Risks of Excessive Pressure
However, too much pressure can degrade battery performance. It may trigger unwanted chemical reactions or accelerate the breakdown of electrode materials. Over time, these effects reduce cycle life and capacity retention. High pressure can also deform electrodes, altering porosity and active surface area, which are key to battery efficiency.
Dendrite Formation Under Pressure
Dendrite growth poses another safety challenge. Moderate pressure may help suppress dendrite formation by ensuring even lithium distribution. But in some conditions, excessive pressure could actually promote dendrite penetration, raising the risk of internal short circuits.
Optimizing Pressure for Safe Operation
To avoid these risks, engineers must carefully manage pressure during both manufacturing and battery use. This involves developing robust materials that tolerate specific pressure ranges and designing pressure control systems that adjust dynamically. Advanced monitoring tools can also help detect early signs of mechanical stress or failure.
Need for Comprehensive Testing
Thorough testing under varied pressure conditions is essential. Researchers need to explore how pressure impacts performance over time and under real-world use. These insights will support safer designs and help unlock the full potential of pressure-enhanced lithium dealloying.
Environmental Impact of Solid-State Battery Production
Solid-state batteries promise to transform the energy storage landscape, but their production raises serious environmental concerns. As global demand grows, manufacturers must address the ecological footprint of every stage in the production process.
Raw Material Extraction and Processing
One of the biggest environmental challenges comes from raw material extraction. Solid-state batteries rely heavily on lithium, which requires extensive mining. Lithium mining can damage ecosystems through soil degradation, water pollution, and habitat destruction, especially in sensitive regions. Other critical materials, such as ceramic electrolytes and specialized polymers, also involve energy-intensive extraction and processing methods. These processes may release harmful chemicals and consume large amounts of energy.
High-Energy Manufacturing Processes
Producing solid-state batteries requires high-temperature sintering and tightly controlled atmospheric conditions. These steps consume significant energy and may release greenhouse gases if powered by fossil fuels. Facilities often rely on clean rooms and advanced manufacturing tools, which further increase energy consumption and carbon emissions unless powered by renewable sources.
Water Use and Local Impact
Manufacturing solid-state batteries also demands large quantities of ultra-pure water for cleaning and processing. This can stress local water supplies, particularly in drought-prone areas. Without efficient recycling systems, water usage can negatively impact surrounding communities and ecosystems. Manufacturers must install closed-loop water systems to minimize this risk.
Waste Generation and Disposal
Waste management remains another key concern. Production may generate chemical byproducts, material waste, and packaging debris. Without proper handling, these waste streams can contribute to environmental degradation. Therefore, companies must adopt recycling, waste reduction, and hazardous material disposal strategies to reduce their environmental impact.
Environmental Advantages of Solid-State Batteries
Despite these concerns, solid-state batteries offer several long-term environmental benefits. They generally last longer than traditional lithium-ion batteries, reducing the need for frequent replacements. Their stable chemical structure also lowers the risk of fires, leaks, and environmental contamination. In the future, researchers aim to make solid-state batteries easier to recycle, which could significantly reduce their end-of-life environmental burden.
Sustainable Solutions for Future Production
To ensure a sustainable future, manufacturers must focus on eco-conscious production practices. This includes using renewable energy to power factories, designing batteries for end-of-life recyclability, and improving the efficiency of raw material extraction. Additionally, closed-loop water systems and zero-waste manufacturing goals should become industry standards.
To get detailed scientific explanations of Pressure on Lithium Dealloying, try Patsnap Eureka.
