
Lithium Dealloying Background and Objectives
Lithium dealloying, a critical process in advanced battery systems, has gained significant attention in recent years due to its potential to revolutionize energy storage technologies. This phenomenon involves the extraction of lithium from electrode materials, which plays a crucial role in the performance and longevity of both solid-state and liquid electrolyte batteries. The evolution of lithium dealloying research can be traced back to the early development of lithium-ion batteries in the 1970s, with substantial progress made in understanding the underlying mechanisms and optimizing the process over the past few decades.
Diving into the effects of pressure on lithium dealloying in solid-state systems? Eureka Technical Q&A connects you with materials science and electrochemistry experts who can help interpret data, explain phase behavior, and guide you through complex research questions—making high-level battery science more accessible and actionable.
The primary objective of this research is to explore the pressure-driven lithium dealloying behavior in advanced solid-state and liquid electrolyte battery systems. By investigating the effects of pressure on the dealloying process, we aim to uncover new insights that could lead to improved battery performance, increased energy density, and enhanced cycling stability. This research is particularly relevant in the context of the growing demand for high-performance energy storage solutions in various applications, including electric vehicles, renewable energy integration, and portable electronics.
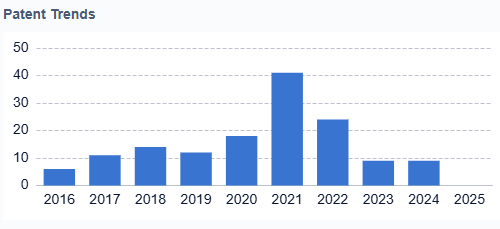
The pressure-driven approach to lithium dealloying represents a novel direction in battery research, as it introduces an additional parameter that can be manipulated to control the dealloying process. Traditional studies have primarily focused on factors such as temperature, current density, and electrolyte composition. By incorporating pressure as a variable, we open up new possibilities for fine-tuning the dealloying behavior and potentially overcoming some of the limitations associated with conventional battery systems.
This research aims to address several key questions, including: How does applied pressure influence the kinetics and thermodynamics of lithium dealloying? What are the structural and morphological changes that occur in electrode materials under pressure-driven dealloying conditions? How can pressure be utilized to enhance the efficiency and reversibility of the dealloying process in both solid-state and liquid electrolyte systems?
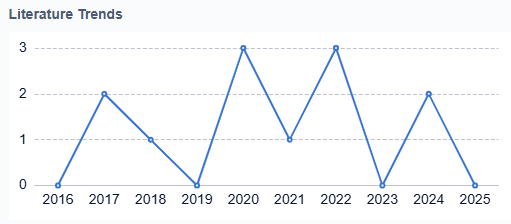
By exploring these questions, we hope to contribute to the development of next-generation battery technologies that offer improved performance, longer lifespans, and enhanced safety. The insights gained from this research could potentially lead to the design of novel electrode materials and battery architectures that leverage pressure-driven dealloying to achieve superior energy storage capabilities. Furthermore, this study may pave the way for new manufacturing techniques and operational strategies that optimize battery performance through controlled pressure application.
Market Analysis for Advanced Battery Systems
The market for advanced battery systems, particularly those incorporating pressure-driven lithium dealloying behavior, is experiencing significant growth and transformation. This trend is driven by the increasing demand for high-performance energy storage solutions across various sectors, including electric vehicles, renewable energy integration, and portable electronics. The global lithium-ion battery market, which encompasses these advanced systems, is projected to reach $129.3 billion by 2027, with a compound annual growth rate (CAGR) of 18.0% from 2020 to 2027.
The automotive industry is a key driver of this market growth, with electric vehicle (EV) adoption accelerating worldwide. As governments implement stricter emissions regulations and offer incentives for EV purchases, the demand for advanced battery systems that can provide longer range, faster charging, and improved safety is surging. The pressure-driven lithium dealloying technology shows promise in addressing these requirements, potentially offering higher energy density and enhanced stability compared to conventional lithium-ion batteries.
In the renewable energy sector, the need for efficient and reliable energy storage solutions is growing as more countries transition to clean energy sources. Advanced battery systems that can effectively manage the intermittent nature of renewable energy generation are in high demand. The pressure-driven lithium dealloying technology could play a crucial role in developing grid-scale storage solutions that offer improved performance and longevity.
The consumer electronics market is another significant driver for advanced battery systems. As devices become more powerful and feature-rich, the demand for batteries with higher capacity, faster charging, and longer lifespan increases. The potential for pressure-driven lithium dealloying to enhance these characteristics makes it an attractive technology for smartphone, laptop, and wearable device manufacturers.
Market analysis indicates that Asia-Pacific is the largest and fastest-growing market for advanced battery systems, driven by the presence of major battery manufacturers and the rapid adoption of electric vehicles in countries like China, Japan, and South Korea. North America and Europe are also significant markets, with increasing investments in research and development of next-generation battery technologies.
The competitive landscape in this market is intense, with established battery manufacturers, automotive companies, and technology giants all vying for a share of the growing market. Start-ups and research institutions are also playing a crucial role in developing and commercializing innovative battery technologies, including those based on pressure-driven lithium dealloying.
Current Challenges in Pressure-Driven Lithium Dealloying
Pressure-driven lithium dealloying in advanced battery systems faces several significant challenges that hinder its widespread implementation and optimization. One of the primary obstacles is the complex interplay between mechanical stress and electrochemical processes. As lithium is extracted from the electrode material, it induces structural changes and volume expansion, leading to mechanical degradation and potential failure of the electrode. This phenomenon is particularly pronounced in solid-state batteries, where the rigid nature of the electrolyte exacerbates the stress-induced damage. Moreover, the heterogeneous distribution of pressure within the battery cell can result in non-uniform lithium extraction, compromising the overall efficiency and cycle life of the battery.
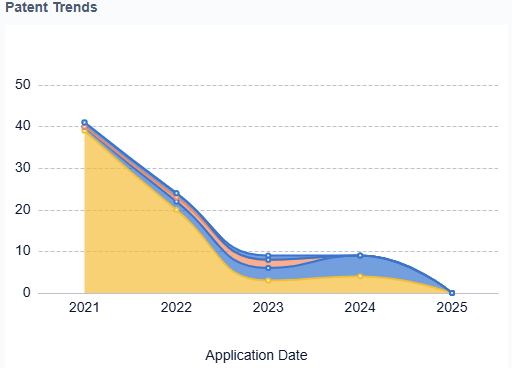
Another critical challenge lies in the limited understanding of the pressure-driven lithium transport mechanisms at the nanoscale. The intricate relationship between applied pressure, lithium diffusion kinetics, and interfacial reactions remains poorly understood, making it difficult to optimize the dealloying process. This knowledge gap hampers the development of predictive models and design strategies for pressure-driven lithium extraction systems. Additionally, the high pressures required for efficient dealloying can lead to undesirable side reactions, such as electrolyte decomposition or the formation of passivation layers, which further complicate the process and reduce overall battery performance.
The scalability and practical implementation of pressure-driven lithium dealloying techniques also present significant hurdles. Maintaining uniform pressure distribution across large-format batteries while ensuring safety and reliability remains a formidable engineering challenge. Furthermore, the integration of pressure-application mechanisms into existing battery manufacturing processes requires substantial modifications to current production lines, potentially increasing costs and complexity.
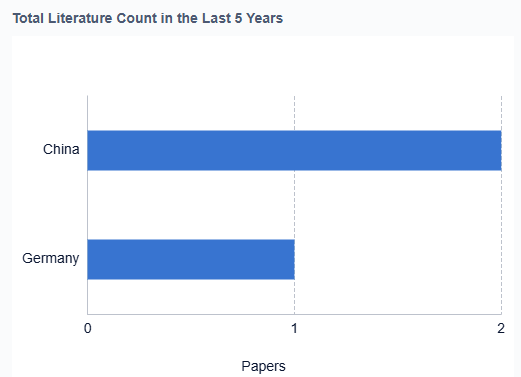
Lastly, the long-term stability and cyclability of pressure-driven lithium dealloying systems are areas of concern. Repeated pressure cycling can lead to fatigue and degradation of both electrode and electrolyte materials, potentially shortening the lifespan of the battery. Addressing these challenges requires a multidisciplinary approach, combining advanced materials science, electrochemistry, and mechanical engineering to develop innovative solutions that can harness the full potential of pressure-driven lithium dealloying in next-generation battery systems.
Evolution of Lithium Dealloying Technologies
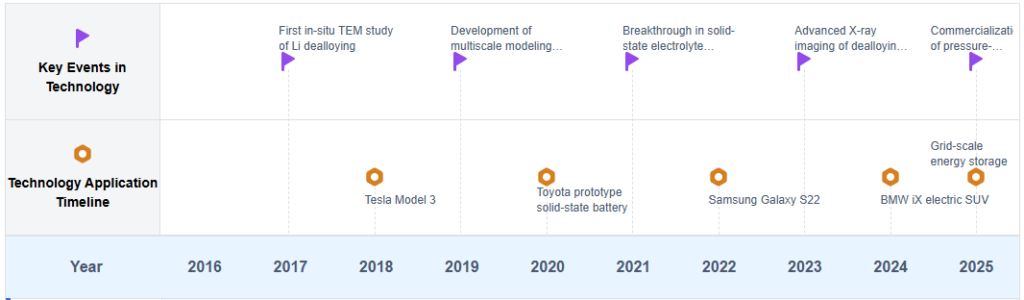
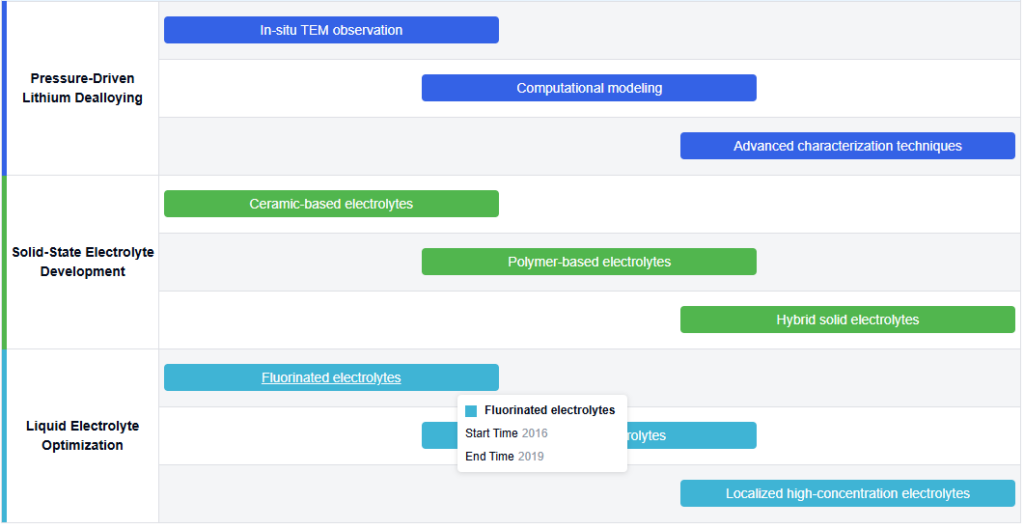
Key Players in Advanced Battery Research
The research on pressure-driven lithium dealloying behavior in advanced solid-state and liquid electrolyte battery systems is at a critical juncture in the industry’s development. The market for advanced battery technologies is rapidly expanding, driven by the growing demand for electric vehicles and renewable energy storage solutions. Companies like QuantumScape, Panasonic, and Samsung Electronics are at the forefront of this technology, investing heavily in research and development. The technical maturity varies, with solid-state batteries still in the early stages of commercialization, while liquid electrolyte systems are more established. Universities and research institutions, such as the University of Maryland and Sichuan University, are contributing significantly to the fundamental understanding of these processes, indicating a collaborative ecosystem between academia and industry in advancing this crucial technology.
QuantumScape Corp.
Technical Solution
QuantumScape is developing solid-state lithium-metal batteries that utilize a ceramic separator. Their technology focuses on a pressure-driven lithium dealloying process, where lithium metal is deposited on the anode during charging and stripped away during discharging. This process is facilitated by the ceramic separator, which allows for the smooth passage of lithium ions while preventing dendrite formation. The company’s approach aims to significantly increase energy density and improve safety compared to conventional lithium-ion batteries.
Strengths: High energy density, improved safety, and potential for fast charging.
Weaknesses: Challenges in scaling up production and ensuring long-term cycling stability.
Panasonic Holdings Corp.
Technical Solution
Panasonic is researching advanced solid-state battery technologies, including pressure-driven lithium dealloying behavior. Their approach involves developing composite electrolytes that combine inorganic and organic materials to enhance ionic conductivity and mechanical stability. The company is exploring various pressure application methods during battery operation to optimize the lithium dealloying process, aiming to improve overall battery performance and longevity.
Strengths: Extensive experience in battery manufacturing and strong R&D capabilities.
Weaknesses: Potential challenges in transitioning from liquid to solid-state electrolytes at scale.
Samsung Electronics Co., Ltd.
Technical Solution
Samsung is investigating pressure-driven lithium dealloying behavior in both solid-state and advanced liquid electrolyte systems. Their research focuses on developing nanostructured electrode materials that can withstand high pressures during cycling. The company is also exploring the use of pressure-sensitive additives in liquid electrolytes to enhance the dealloying process and improve overall battery performance. Samsung’s approach aims to balance the benefits of solid-state and liquid electrolyte systems.
Strengths: Strong technological expertise and resources for large-scale production.
Weaknesses: Balancing performance improvements with cost-effectiveness in new battery technologies.
Robert Bosch GmbH
Technical Solution
Bosch is researching pressure-driven lithium dealloying behavior in advanced battery systems, focusing on the interface between electrodes and electrolytes. Their approach involves developing novel electrode architectures that can accommodate volume changes during cycling while maintaining optimal pressure distribution. The company is also investigating the use of smart pressure management systems to dynamically adjust internal battery pressure during operation, potentially enhancing the dealloying process and overall battery performance.
Strengths: Extensive experience in automotive systems and integration capabilities.
Weaknesses: Potential challenges in adapting new battery technologies to meet diverse automotive requirements.
Sion Power Corp.
Technical Solution
Sion Power is developing advanced lithium-metal batteries with a focus on pressure-driven lithium dealloying behavior. Their proprietary Licerion® technology combines a lithium metal anode with a high-performance electrolyte system. The company’s research includes optimizing the pressure distribution within the cell to enhance the dealloying process, improve cycling efficiency, and extend battery life. Sion Power is also exploring the use of protective coatings on the lithium metal anode to mitigate unwanted side reactions during the pressure-driven dealloying process.
Strengths: Specialized focus on lithium-metal battery technology and high energy density solutions. Weaknesses: Potential challenges in scaling up production and competing with larger, established battery manufacturers.
Existing Pressure-Driven Dealloying Solutions
Dealloying mechanisms in lithium battery electrodes
Dealloying behavior in lithium battery electrodes involves the selective dissolution of one or more elements from an alloy during charge-discharge cycles. This process can lead to structural changes, affecting the battery’s performance and longevity. Understanding these mechanisms is crucial for developing more stable and efficient electrode materials.
- Dealloying mechanisms in lithium battery electrodes
Dealloying is a significant mechanism affecting the performance and longevity of lithium battery electrodes. This process involves the selective dissolution of one or more components from an alloy, leading to structural changes in the electrode material. Understanding these mechanisms is crucial for developing more stable and efficient lithium battery electrodes. - Impact of dealloying on electrode structure and performance
Dealloying in lithium battery electrodes can lead to significant changes in the electrode structure, including porosity formation, surface area increase, and compositional changes. These structural modifications can affect the electrode’s electrochemical performance, cycling stability, and capacity retention. Understanding these impacts is essential for optimizing electrode design and improving battery life. - Strategies to mitigate dealloying effects
Various strategies have been developed to mitigate the negative effects of dealloying in lithium battery electrodes. These include surface coatings, doping with stabilizing elements, and designing novel alloy compositions that are more resistant to dealloying. Implementing these strategies can lead to improved electrode stability and enhanced battery performance. - In-situ characterization of dealloying behavior
Advanced in-situ characterization techniques are being employed to study dealloying behavior in lithium battery electrodes in real-time. These methods provide valuable insights into the dynamic processes occurring during battery operation, allowing researchers to better understand the mechanisms of dealloying and develop more effective mitigation strategies.
Nanostructured materials to mitigate dealloying effects
Nanostructured materials, such as nanoparticles or nanoporous structures, are being investigated to mitigate the negative effects of dealloying in lithium batteries. These materials can provide better structural stability, enhanced conductivity, and improved lithium-ion diffusion, potentially leading to increased cycle life and performance.Expand
Surface coatings and modifications to prevent dealloying
Various surface coating techniques and modifications are being developed to prevent or reduce dealloying in lithium battery electrodes. These approaches aim to create protective layers that can inhibit the selective dissolution of elements while maintaining good ionic and electronic conductivity.Expand
In-situ characterization of dealloying processes
Advanced in-situ characterization techniques are being employed to study dealloying processes in real-time during battery operation. These methods provide valuable insights into the structural and chemical changes occurring at the electrode-electrolyte interface, helping researchers to better understand and address dealloying-related issues.Expand
Novel alloy compositions for improved dealloying resistance
Researchers are exploring novel alloy compositions and design strategies to enhance the resistance to dealloying in lithium battery electrodes. These efforts focus on optimizing the chemical and physical properties of electrode materials to maintain structural integrity and electrochemical performance over extended cycling.
Core Innovations in Lithium Dealloying Mechanisms
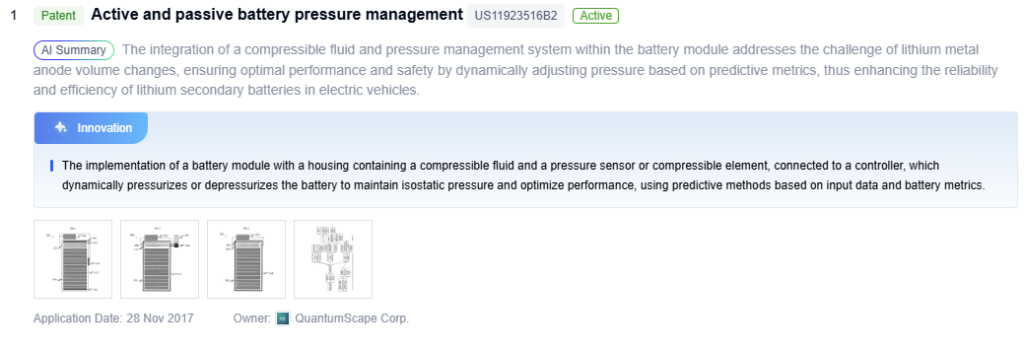
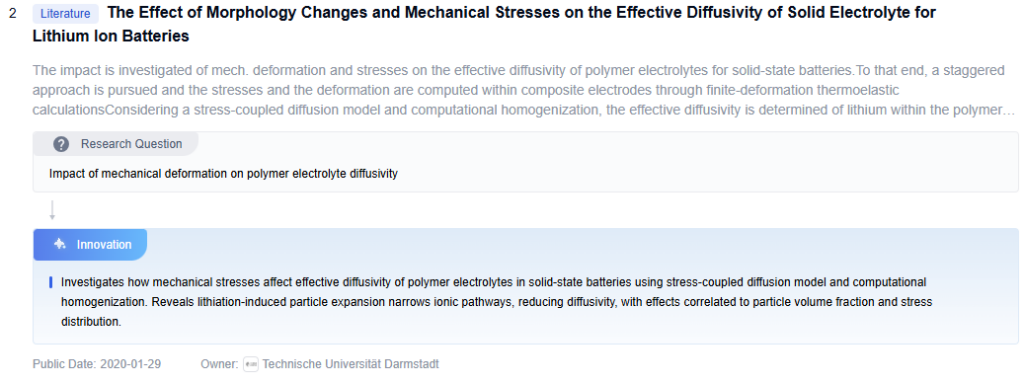
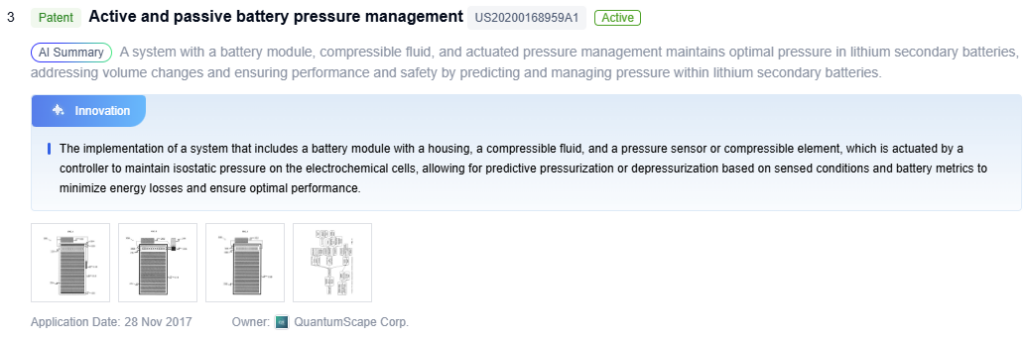
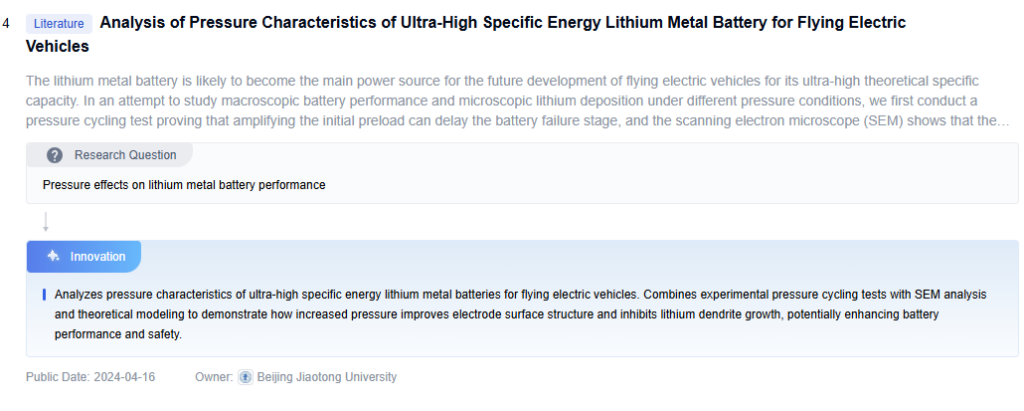
Future Directions in Pressure-Driven Dealloying
Advanced Pressure-Controlled Dealloying Systems
Advanced pressure-controlled dealloying systems are opening new frontiers in battery research and design. These systems aim to precisely manage pressure during the dealloying process in both solid-state and liquid electrolyte batteries. By fine-tuning pressure parameters, researchers can improve battery performance, stability, and design flexibility.
Precision Engineering for Better Control
This approach centers on building sophisticated pressure chambers and microfluidic devices that apply and regulate pressure with high accuracy. These systems include real-time sensors, feedback controls, and even machine learning algorithms to dynamically optimize pressure profiles. With this level of control, scientists can tailor porosity, surface area, and composition of the dealloyed structures for specific applications.
Customizable Pressure Profiles
Researchers can adjust variables like pressure magnitude, duration, and cycling patterns to explore a wide range of dealloying behaviors. This flexibility supports the design of novel electrode architectures that deliver better ionic conductivity, mechanical stability, and electrochemical performance.
Integration with In-Situ Characterization
These advanced systems can work with in-situ tools like X-ray tomography or neutron diffraction. Real-time data from these tools help researchers observe structural changes as they happen. This allows rapid optimization of battery materials and accelerates the development process.
Exploring Innovative Dealloying Media
Beyond pressure alone, these systems can use supercritical fluids or gas-expanded liquids as dealloying media. These substances offer superior diffusion properties, helping to achieve more uniform and controlled dealloying, especially across micro- and nano-scales. This could address common limitations in traditional liquid-based systems, such as uneven ion distribution.
A Path Toward High-Performance Battery Designs
By combining pressure control with advanced electrochemical design, researchers can develop batteries with higher energy density, longer cycle life, and improved overall efficiency. This approach supports a new wave of high-performance, customizable batteries for applications ranging from electric vehicles to grid storage.
Strengths
- Enables precise control of dealloying processes
- Enhances customization of electrode structures
- Improves ionic conductivity and cycling stability
- Supports in-situ analysis and real-time optimization
- Works with both solid-state and liquid electrolyte batteries
Weaknesses
- Requires complex and expensive equipment
- Presents challenges in scaling up for mass production
- Introduces safety concerns due to high-pressure conditions
Safety Considerations in Advanced Battery Systems
Safety remains a top priority in the development of advanced battery technologies. This is especially true for systems involving pressure-driven lithium dealloying, where evolving designs introduce new risks. As solid-state and liquid electrolyte batteries advance, engineers must proactively manage potential hazards.
Solid-State Systems: New Safety Challenges Under Pressure
Solid-state batteries are generally safer than traditional lithium-ion designs. They use solid electrolytes, which reduce risks like leakage and flammability. However, pressure-driven lithium dealloying introduces unique mechanical stresses.
These stresses can trigger dendrite formation, which leads to internal short circuits and potential thermal runaway. Pressure may also damage the interface between electrodes and electrolytes, reducing battery life and stability.
Liquid Electrolyte Systems: Higher Reactivity and Risk
Liquid electrolyte batteries face more complex safety issues. Their use of flammable solvents increases the risk of fires and explosions. Applying pressure during dealloying can worsen this by promoting electrolyte decomposition and gas buildup.
Pressure-induced gas generation may cause cell rupture or even explosions. At the same time, dealloyed lithium can react more easily with the liquid electrolyte, increasing the chance of harmful side reactions.
Strategies to Improve Battery Safety
Researchers are working on several strategies to mitigate these risks. First, they design pressure-resistant battery cells that prevent structural failure. Second, they use advanced separators that block dendrites and support safer operation.
Engineers also incorporate pressure relief valves to release excess gas safely. In parallel, chemists are developing less reactive electrolyte formulas that remain stable under high-pressure conditions.
Battery Management Systems and Real-Time Monitoring
Battery management systems (BMS) play a vital role in maintaining safe performance. These systems monitor pressure, temperature, and voltage in real time. When anomalies appear, the BMS triggers early intervention, preventing accidents before they escalate.
To further improve safety, teams are testing non-destructive methods to assess structural integrity under pressure. These tests ensure that cells maintain their form and function over time.
Establishing Clear Safety Standards
Moving forward, the industry must create specialized safety protocols for pressure-driven dealloying systems. These standards should apply to both solid-state and liquid electrolyte batteries.
Developing these protocols requires collaboration across the battery industry. Researchers, manufacturers, and regulators must work together to define testing methods, response plans, and best practices.
By addressing safety at every stage—from materials to system design—advanced batteries can achieve greater reliability and consumer confidence.
Environmental Impact of Lithium Dealloying Processes
As the demand for lithium-ion batteries grows, researchers must examine the environmental impact of pressure-driven lithium dealloying. While this technique offers benefits for battery performance and lifespan, it also raises key sustainability concerns.
Increased Energy Consumption
One major environmental issue is the higher energy demand during manufacturing. Applying pressure to induce dealloying requires additional energy, especially in solid-state systems. If powered by non-renewable sources, this energy use can lead to increased carbon emissions. In some cases, the added emissions may offset the climate benefits of lithium-ion batteries used in electric vehicles and renewable energy storage.
Water Use and Wastewater Concerns
Water usage is another important factor. Some dealloying processes involve aqueous or liquid-based media, which increase water consumption and generate wastewater. This wastewater may contain lithium, heavy metals, or other contaminants. Without proper treatment, it can threaten local water supplies and surrounding ecosystems.
Raw Material Extraction and Processing
The process may also increase demand for rare materials and specialized chemicals, which could expand mining operations. Mining contributes to habitat destruction, soil degradation, and water pollution, especially in sensitive regions. As a result, lithium dealloying may indirectly amplify the environmental footprint of battery production.
Challenges in Recycling and End-of-Life Management
Pressure-based dealloying can change the structure and composition of electrode materials. These changes may complicate recycling by making it harder to separate and recover valuable metals. If recycling becomes less efficient, more batteries could end up as electronic waste, adding pressure on disposal systems.
Potential Environmental Benefits
Despite these concerns, pressure-driven lithium dealloying offers potential environmental advantages. If the process extends battery lifespan and improves energy density, it could reduce the need for frequent replacements. Fewer replacements would lead to less resource use, lower production emissions, and reduced waste over time.
In addition, more energy-dense batteries require fewer materials per unit of energy, which can reduce the overall material footprint. These gains could help offset some environmental costs during production.
The Need for Life Cycle Analysis
To evaluate the true environmental impact, researchers must conduct a comprehensive life cycle assessment (LCA). This includes every stage—from raw material extraction to manufacturing, use, and recycling. A well-executed LCA can highlight opportunities for environmental improvement and support the development of greener battery technologies.
By addressing these impacts early, the industry can design dealloying techniques that balance performance gains with sustainability goals, ensuring lithium batteries remain a clean energy solution.
To get detailed scientific explanations of Pressure-Driven Lithium Dealloying, try Patsnap Eureka.
