
Galvanic cells, also known as voltaic cells, are electrochemical cells that convert chemical energy into electrical energy through spontaneous redox reactions. This article explores what galvanic cells are, how they work, what components they require, and how to calculate cell voltage.
Curious about how galvanic cells generate voltage or how redox reactions power electrochemical systems? Eureka Technical Q&A connects you with chemistry experts who can clarify concepts, explain cell components, and walk you through real-world applications—making complex electrochemistry easier to understand and apply.
What Is a Galvanic Cell?
A galvanic cell is a type of electrochemical cell that produces electricity as a result of a spontaneous redox (oxidation-reduction) reaction. Electrons flow through an external circuit from the anode (oxidation site) to the cathode (reduction site), generating a measurable electric current.
Galvanic cells are the fundamental working principle behind batteries, making them essential in both everyday devices and industrial energy storage.
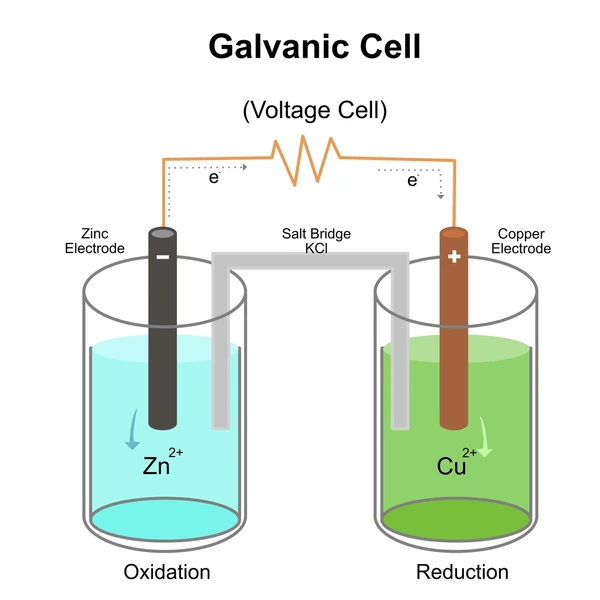
Key Components of a Galvanic Cell
Each galvanic cell has several crucial parts that enable electron transfer and energy conversion:
1. Anode
- Site of oxidation (loss of electrons)
- Electrons are released into the external circuit
- Marked as the negative terminal
2. Cathode
- Site of reduction (gain of electrons)
- Electrons are accepted from the circuit
- Marked as the positive terminal
3. Electrodes
- Solid conductors (usually metal) where oxidation/reduction occurs
- Examples: Zinc, copper, platinum
4. Electrolyte Solution
- Contains ions that complete the internal circuit and support redox reactions
- Often aqueous metal salt solutions (e.g., CuSO₄, ZnSO₄)
5. Salt Bridge
- A tube or membrane containing a neutral salt solution (e.g., KNO₃)
- Maintains electrical neutrality by allowing ion flow between half-cells
- Prevents the buildup of charge that would stop the reaction
6. External Wire
- Allows electron flow from anode to cathode
- May connect to a voltmeter or electrical load
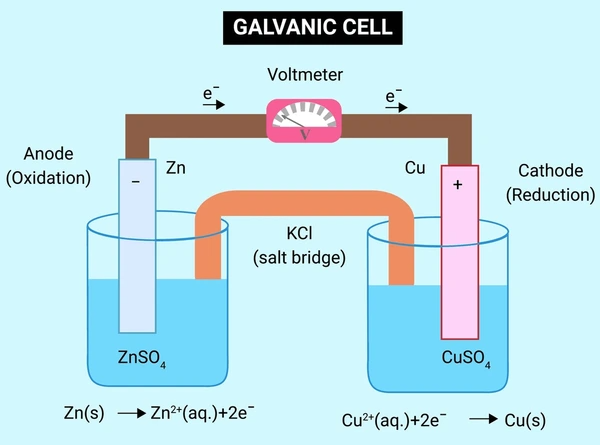
How a Galvanic Cell Works: Step-by-Step
Let’s walk through the process using a zinc-copper (Zn-Cu) galvanic cell as an example:
1. Oxidation at the Anode
Zinc metal loses electrons and becomes zinc ions
Zn(s) → Zn²⁺(aq) + 2e⁻
2. Electron Flow Through the Wire
Electrons travel through the external circuit from zinc (anode) to copper (cathode).
3. Reduction at the Cathode
Copper ions in the solution gain electrons and become solid copper
Cu²⁺(aq) + 2e⁻ → Cu(s)
4. Ion Flow Through the Salt Bridge
- Anions move toward the anode to balance positive Zn²⁺ ions.
- Cations move toward the cathode to replace Cu²⁺ being reduced.
Result: Electrical energy is generated from a spontaneous chemical reaction.
Half-Reactions and Overall Cell Reaction
For the Zn-Cu galvanic cell:
Anode (oxidation):
Zn(s) → Zn²⁺(aq) + 2e⁻
Cathode (reduction):
Cu²⁺(aq) + 2e⁻ → Cu(s)
Overall Cell Reaction:
Zn(s) + Cu²⁺(aq) → Zn²⁺(aq) + Cu(s)
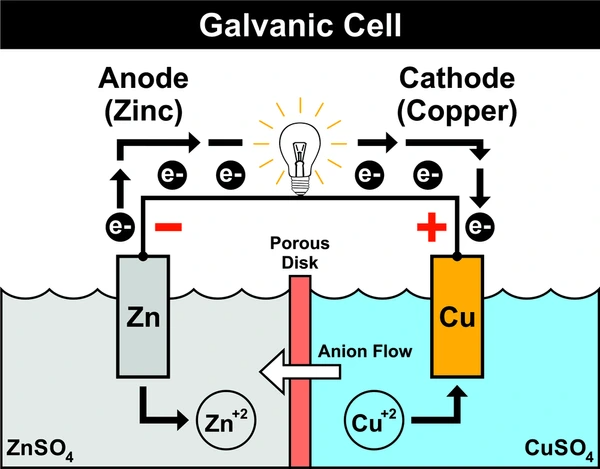
Cell Notation (Cell Diagram)
Galvanic cells are commonly written in shorthand cell notation:
Zn(s) | Zn²⁺(aq) || Cu²⁺(aq) | Cu(s)
- Single vertical line (|) separates different phases.
- Double vertical line (||) represents the salt bridge.
Calculating Cell Voltage (Standard Electromotive Force, E°cell)
The standard cell potential (E°cell) is calculated from the standard electrode potentials:
E°cell = E°cathode – E°anode
For Zn/Cu cell:
- E°(Cu²⁺/Cu) = +0.34 V
- E°(Zn²⁺/Zn) = –0.76 V
E°cell = 0.34 – (–0.76) = +1.10 V
A positive voltage indicates a spontaneous reaction, confirming the cell works as a galvanic cell.
Factors That Affect Galvanic Cell Voltage
- Concentration of solutions
- Non-standard conditions change voltage (use the Nernst equation).
- Temperature
- Affects reaction kinetics and equilibrium.
- Electrode surface area
- Larger area can increase current, not voltage.
- Salt bridge integrity
- A broken salt bridge will stop ion flow and halt the cell reaction.
Real-Life Applications of Galvanic Cells
Batteries
Batteries are the most common and practical use of galvanic cells. They power countless everyday devices, from flashlights to smartphones. There are two main types: primary batteries, which are disposable, and secondary batteries, which are rechargeable. Both types rely on galvanic reactions to deliver steady power. Batteries also support critical systems in electric vehicles, medical tools, and backup power supplies.
Wearable Devices
Galvanic cells power many wearable electronics by providing a lightweight and reliable voltage source. Some advanced designs use the body’s sweat as an electrolyte to generate energy. These self-powered cells are ideal for small devices like fitness trackers or medical monitors. As wearable tech advances, these innovative power sources will become even more useful.
Energy Harvesting
Researchers also use galvanic cells for energy harvesting in ingestible medical devices. These cells activate upon contact with stomach or intestinal fluids. Once triggered, they generate power for a few days, enough to support sensors that monitor body temperature or deliver drugs. This application shows how galvanic cells enable self-powered medical technology.
Electrochemical Sensors
Galvanic cells serve as effective components in electrochemical sensors. These devices detect specific chemicals by converting concentration changes into electrical signals. For example, a galvanic cell sensor can detect melamine in milk by measuring voltage shifts. The signal is then processed by a microcontroller for data analysis.
Experimental Thermodynamic Measurements
Scientists use galvanic cells in thermodynamic studies to explore material properties. By measuring electromotive force, researchers determine characteristics like Gibbs free energy. This method involves ion-conducting electrolytes—either liquid or solid—and is widely used to study metals and ceramics in research labs.
Low-Cost Educational Tools
Galvanic cells also play a key role in education. Students and teachers use simple setups made from household materials to study redox reactions. These low-cost educational tools help demonstrate core principles in electrochemistry and make classroom learning more engaging and hands-on.
Comparing Galvanic vs. Electrolytic Cells
Definition and Principle
Galvanic cells convert chemical energy into electrical energy through spontaneous redox reactions. These reactions occur naturally and require no external power. As a result, galvanic cells generate current that powers devices or performs useful work.
On the other hand, electrolytic cells do the opposite. They use electrical energy from an external source to drive non-spontaneous chemical reactions. In this way, electrolytic cells reverse the natural direction of redox processes found in galvanic cells.
Components and Operation
Both galvanic and electrolytic cells share key components. Each includes an anode, a cathode, and an electrolyte. However, they operate in different ways.
In a galvanic cell, oxidation happens at the anode. The released electrons travel through an external circuit to the cathode, where reduction occurs. This flow of electrons generates usable electrical energy.
In an electrolytic cell, external voltage pushes electrons in the opposite direction. This forces oxidation at the anode and reduction at the cathode, even though the reactions are non-spontaneous.
Applications
Galvanic cells are widely used in batteries and fuel cells. These power portable electronics, electric vehicles, and other devices that need a constant energy supply.
In contrast, electrolytic cells serve different purposes. Industries use them for electrolysis to produce or separate chemicals. A common example is splitting water into hydrogen and oxygen using electricity.
Key Differences
The main difference lies in how each cell functions. Galvanic cells create electrical energy from chemical reactions. Electrolytic cells use electrical energy to trigger chemical changes.
In a galvanic cell, the anode carries a negative charge, and the cathode is positive. In an electrolytic cell, the charges switch due to the applied voltage. The anode becomes positive, and the cathode becomes negative.
Feature | Galvanic Cell | Electrolytic Cell |
---|---|---|
Energy Conversion | Chemical → Electrical | Electrical → Chemical |
Reaction Type | Spontaneous | Non-spontaneous |
Anode Sign | Negative | Positive |
Cathode Sign | Positive | Negative |
Example | Battery | Electroplating |
Conclusion
Galvanic cells are a foundational concept in electrochemistry, powering everything from flashlights to cars. By converting chemical energy into electrical energy via spontaneous redox reactions, these systems demonstrate the practical application of chemistry in real-world technology. Understanding the structure, function, and calculation of voltage in galvanic cells empowers students and professionals to explore deeper electrochemical systems.
FAQs
They are the same; both terms refer to electrochemical cells that generate electricity from spontaneous redox reactions.
Because oxidation occurs at the anode, it releases electrons, making it the source (negative terminal).
It maintains charge neutrality by allowing ion exchange, which sustains the flow of electrons.
No. Once reactants are consumed or ions accumulate imbalances, the reaction stops.
It means the redox reaction is spontaneous under standard conditions.
To get detailed scientific explanations of galvanic cell, try Patsnap Eureka.
