Molecular Engineering Techniques For Developing Advanced Materials
Molecular Engineering Techniques and Goals
By analyzing the evolution of this technology domain, the report seeks to shed light on the anticipated trajectory of future advancements, highlighting promising research directions and potential disruptive innovations that could revolutionize the field. This analysis will serve as a foundation for formulating strategic recommendations and insights to guide long-term planning and decision-making processes within the organization.
Market Demand for Advanced Materials
- Growing Demand for Advanced Materials
Advanced materials are crucial for driving innovation across various industries, including electronics, energy, aerospace, and healthcare. The demand for these materials is fueled by the need for improved performance, energy efficiency, and sustainability. - Emerging Applications
Emerging applications such as flexible electronics, lightweight composites, energy storage systems, and biomedical devices are driving the demand for advanced materials with unique properties and functionalities. - Technological Advancements
Advancements in nanotechnology, materials science, and manufacturing processes have enabled the development of advanced materials with tailored properties, leading to increased adoption across diverse sectors. - Environmental and Sustainability Factors
The growing emphasis on environmental sustainability and resource conservation is driving the demand for advanced materials that are lightweight, durable, and recyclable, reducing the overall environmental impact. - Market Growth Projections
The global market for advanced materials is projected to experience significant growth, driven by increasing investments in research and development, as well as the adoption of these materials across various industries.
Current State and Challenges in Molecular Engineering
- Technological Maturity
Molecular engineering techniques have advanced significantly, enabling precise control over material properties at the molecular level. However, challenges remain in scaling up production and ensuring consistent quality. - Computational Limitations
Current computational methods struggle to accurately model complex molecular interactions and predict material behavior, hindering the design and optimization of advanced materials. - Characterization Challenges
Characterizing the structure and properties of engineered materials at the molecular scale remains a significant challenge, requiring advanced analytical techniques and instrumentation. - Interdisciplinary Collaboration
Molecular engineering requires collaboration across disciplines, including chemistry, physics, materials science, and engineering, which can be challenging due to differing perspectives and approaches.
Evolution of Molecular Engineering Technologies
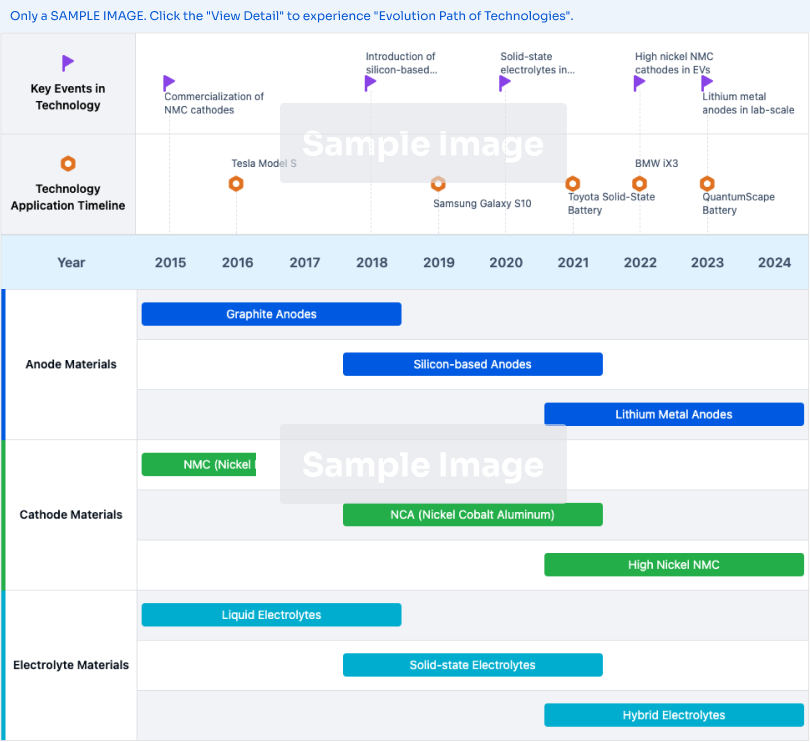
Existing Solutions in Molecular Engineering
01 Material Synthesis
Techniques for designing and constructing molecular structures, controlling molecular interactions, and optimizing synthesis processes to engineer molecules for synthesizing new materials with desired properties.- Material Synthesis: Techniques for designing and constructing molecular structures, controlling molecular interactions, and assembling molecules into larger structures or devices to engineer molecules for synthesizing new materials with desired properties.
- Energy Applications: Approaches for designing molecular structures for efficient energy transfer, catalysis, or photovoltaic processes to engineer molecules for energy-related applications, such as energy storage, energy conversion, and energy transport.
- Biological and Medical Applications: Techniques for designing molecular structures for specific biological interactions, targeting, or therapeutic effects to engineer molecules for biological and medical applications, such as drug delivery, biosensing, and tissue engineering.
- Electronic and Optoelectronic Devices: Approaches for designing molecular structures for efficient charge transport, light emission, or signal processing to engineer molecules for electronic and optoelectronic devices, such as molecular electronics, organic semiconductors, and photonic devices.
- Catalysis and Chemical Processes: Techniques for designing molecular structures for specific catalytic activities, molecular recognition, or selective transport to engineer molecules for catalytic and chemical processes, such as catalysis, chemical sensing, and chemical separations.
02 Drug Delivery
Approaches for modifying molecular structures and incorporating functional groups to engineer molecules for improving drug delivery systems, such as targeted delivery, controlled release, and enhanced bioavailability.03 Catalysis
Techniques for designing molecular catalysts, modifying catalyst structures, and optimizing catalytic processes to engineer molecules for enhancing catalytic activity and selectivity.04 Energy Storage and Conversion
Approaches for designing molecular structures for efficient energy storage, conversion, and transport to engineer molecules for improving energy storage and conversion systems, such as batteries, fuel cells, and solar cells.05 Sensing and Detection
Techniques for designing molecular probes, modifying molecular recognition properties, and optimizing signal transduction mechanisms to engineer molecules for developing sensitive and selective sensors and detection systems.
Key Players in Advanced Materials Industry
Dow Global Technologies LLC
International Business Machines Corp.
Core Innovations in Molecular Engineering
- Developing computational methods for modeling molecular structures
- Improving accuracy and efficiency of molecular structure simulations
- Enabling design and optimization of advanced materials through molecular modeling
Regulatory Landscape for Molecular Engineering Techniques
The regulatory landscape for molecular engineering techniques aimed at developing advanced materials is largely governed by national and international guidelines and policies. Key considerations include biosafety protocols for handling genetically modified organisms, environmental impact assessments, and ethical oversight for emerging technologies. Regulatory bodies like the US Environmental Protection Agency (EPA) and the European Chemicals Agency (ECHA) play a crucial role in establishing safety standards and monitoring compliance. Intellectual property rights and technology transfer agreements also influence the commercialization and dissemination of these techniques. As this field rapidly evolves, a collaborative effort between policymakers, researchers, and industry stakeholders is necessary to ensure responsible innovation while fostering scientific progress and economic growth.
Environmental Impact of Molecular Engineering Techniques For Developing Advanced Materials
Molecular engineering techniques for developing advanced materials, while offering significant potential benefits, raise concerns about their environmental impact. These techniques involve manipulating matter at the molecular level, potentially leading to the release of novel substances or nanomaterials into the environment. Assessing and mitigating the environmental risks associated with these processes is crucial. Key considerations include the potential for bioaccumulation, ecosystem disruption, and long-term effects on biodiversity. Additionally, the energy consumption and carbon footprint of these techniques should be evaluated. A comprehensive risk assessment framework, coupled with robust regulations and responsible practices, is essential to ensure the sustainable development and application of molecular engineering in materials science.