Integrated overdrive and overvoltage protection device
A protection device, overvoltage technology, applied in the direction of emergency protection circuit device for limiting overcurrent/overvoltage, protection reacting to overvoltage, emergency protection circuit device, etc., can solve problems such as damage
- Summary
- Abstract
- Description
- Claims
- Application Information
AI Technical Summary
Problems solved by technology
Method used
Image
Examples
Embodiment Construction
[0023] figure 1 is a block diagram illustrating the input power protection device 100 . like figure 1 As shown in , input power protection device 100 is coupled to overcurrent protection portion 110 (which may include one or more devices), and overcurrent protection portion 110 is coupled to overvoltage protection portion 130 (which may include one or more devices). In addition, the input power protection device 100 includes a control circuit 120 coupled to the overcurrent protection part 110 and the overvoltage protection part 130 . Accordingly, the overcurrent protection portion 110 may be coupled to the overvoltage protection portion 130 when coupled via the control circuit 120 . In some embodiments, the overcurrent protection portion 110 and the overvoltage protection portion 130 (and / or portions thereof) may collectively be referred to as components of the input power protection device 100 .
[0024] Input power protection device 100 is configured to provide power pr...
PUM
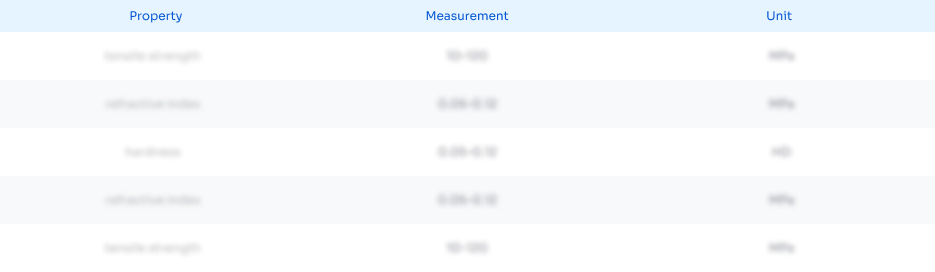
Abstract
Description
Claims
Application Information
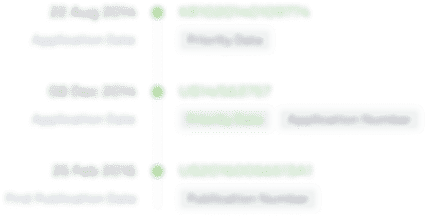
- Generate Ideas
- Intellectual Property
- Life Sciences
- Materials
- Tech Scout
- Unparalleled Data Quality
- Higher Quality Content
- 60% Fewer Hallucinations
Browse by: Latest US Patents, China's latest patents, Technical Efficacy Thesaurus, Application Domain, Technology Topic, Popular Technical Reports.
© 2025 PatSnap. All rights reserved.Legal|Privacy policy|Modern Slavery Act Transparency Statement|Sitemap|About US| Contact US: help@patsnap.com