Hinge concealed micro electromechanical device
A technology of micro-electromechanical devices and electrodes, which is applied in the field of forming integrated devices such as semiconductor devices, and can solve problems such as hinge elasticity, mirrors that cannot be completely flat, and mirror heights that are different
- Summary
- Abstract
- Description
- Claims
- Application Information
AI Technical Summary
Problems solved by technology
Method used
Image
Examples
Embodiment Construction
[0017] For the purposes of this application, the terms "wafer" and "substrate" are used interchangeably, differing only in their dimensions.
[0018] The method according to the invention is particularly suitable for producing micromirror spatial light modulators. However, it can be applied to various microelectromechanical devices, thermal detectors and non-thermal detector devices such as quantum well detectors, pyroelectric detectors, bolometers, etc., but not limited thereto. The invention is particularly applicable when a structure (eg micromirror array) cannot be directly processed / patterned / deposited on a substrate with another structure (eg steering electronics). This is the case, for example, if the structures provided on said substrate are sensitive to the process temperature of the processing of the structures to be provided thereon, or when the substrate is polycrystalline and the components grown on the substrate must be monocrystalline.
[0019] Fig. 1 schematic...
PUM
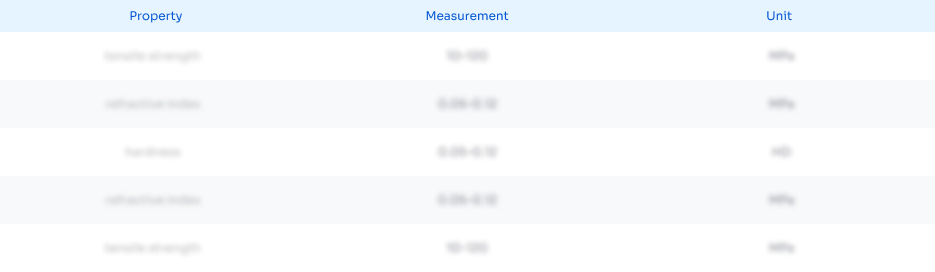
Abstract
Description
Claims
Application Information
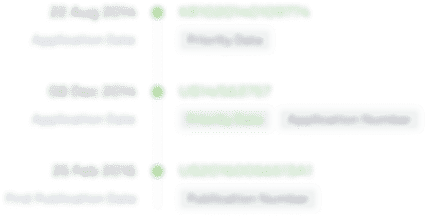
- Generate Ideas
- Intellectual Property
- Life Sciences
- Materials
- Tech Scout
- Unparalleled Data Quality
- Higher Quality Content
- 60% Fewer Hallucinations
Browse by: Latest US Patents, China's latest patents, Technical Efficacy Thesaurus, Application Domain, Technology Topic, Popular Technical Reports.
© 2025 PatSnap. All rights reserved.Legal|Privacy policy|Modern Slavery Act Transparency Statement|Sitemap|About US| Contact US: help@patsnap.com