Front anti-collision beam assembly lightweight designing method based on independent assessment working condition
A light-weight design and front anti-collision beam technology, which is applied in the direction of calculation, instrumentation, and geometric CAD, can solve the problems of the front anti-collision beam assembly that lacks consideration of the performance of the performance verification, so as to shorten the development cycle and improve development. Efficiency and the effect of reducing development costs
- Summary
- Abstract
- Description
- Claims
- Application Information
AI Technical Summary
Problems solved by technology
Method used
Image
Examples
Embodiment
[0154] The present invention combines the specific data of a certain passenger car as the basic vehicle model to introduce the establishment of independent evaluation conditions of the front anti-collision beam assembly proposed by the present invention and to verify the validity of the independent conditions, and to use the independent conditions to carry out front anti-collision beam assembly. The process of lightweight design of the impact beam assembly.
[0155] 1. Select a variety of collision conditions
[0156] The front anti-collision beam assembly of the basic vehicle is designed respectively by selecting three working conditions of the frontal full-width high-speed collision condition, the frontal 40% overlap low-speed collision condition and the static pressure condition.
[0157] 2. Determine the performance target of the front anti-collision beam assembly
[0158] 1) Determine the lightweight design performance target of the front anti-collision beam assembly bas...
PUM
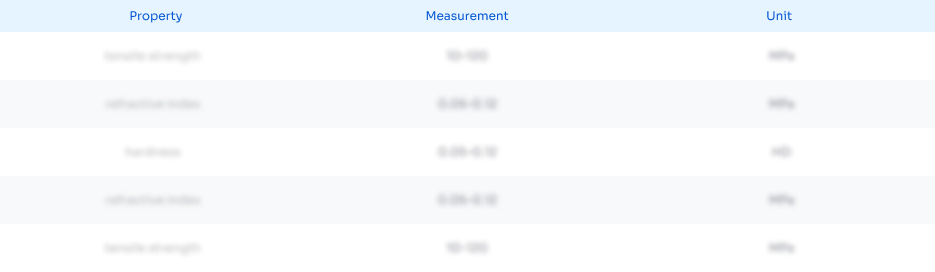
Abstract
Description
Claims
Application Information
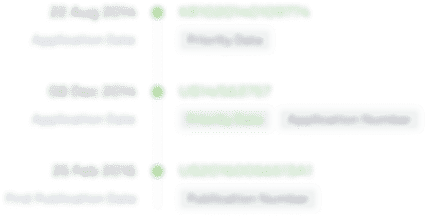
- R&D Engineer
- R&D Manager
- IP Professional
- Industry Leading Data Capabilities
- Powerful AI technology
- Patent DNA Extraction
Browse by: Latest US Patents, China's latest patents, Technical Efficacy Thesaurus, Application Domain, Technology Topic, Popular Technical Reports.
© 2024 PatSnap. All rights reserved.Legal|Privacy policy|Modern Slavery Act Transparency Statement|Sitemap|About US| Contact US: help@patsnap.com