Rotating blade and air foil with structure for increasing flow rate
A technology of rotating blades and airfoils, which is used in the components of pumping devices for elastic fluids, mechanical equipment, machines/engines, etc., to achieve the effect of high revolutions and high energy conversion efficiency
- Summary
- Abstract
- Description
- Claims
- Application Information
AI Technical Summary
Problems solved by technology
Method used
Image
Examples
Embodiment Construction
[0023] Refer to the accompanying drawings below Figure 1 to Figure 6 , to further describe preferred embodiments of the present invention in detail. Multiple embodiments in the present invention can have multiple modifications, and the scope of the present invention is not limited by the multiple embodiments described below, and is only used to provide information for those of ordinary skill in the technical field to which multiple embodiments in the present invention belong. More detailed instructions. Therefore, in order to more clearly emphasize and illustrate the problem, the shapes of the various components shown in the drawings may be exaggerated.
[0024] figure 1 Shown is the situation where the propeller is placed in a wind tunnel. The wind tunnel 10 is arranged laterally, a fan (fan) is installed at the right end of the wind tunnel, and an exhaust port is formed at the left end of the wind tunnel. The fluid flow (V1) provided by the fan passes through the (V) pr...
PUM
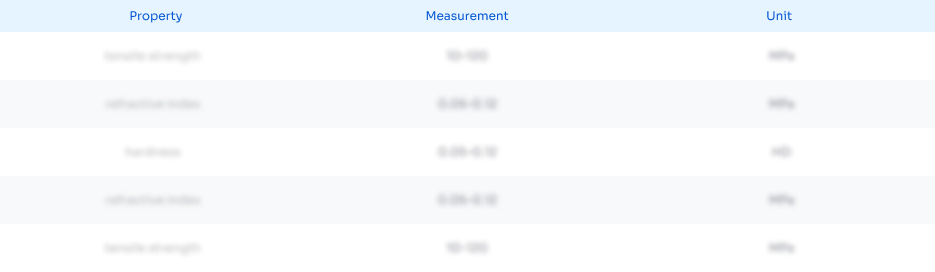
Abstract
Description
Claims
Application Information
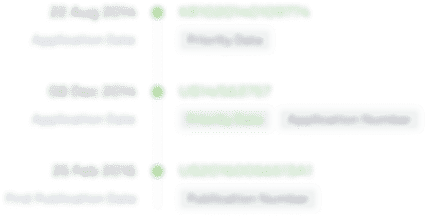
- R&D Engineer
- R&D Manager
- IP Professional
- Industry Leading Data Capabilities
- Powerful AI technology
- Patent DNA Extraction
Browse by: Latest US Patents, China's latest patents, Technical Efficacy Thesaurus, Application Domain, Technology Topic, Popular Technical Reports.
© 2024 PatSnap. All rights reserved.Legal|Privacy policy|Modern Slavery Act Transparency Statement|Sitemap|About US| Contact US: help@patsnap.com