Refrigeration system using non-azeotropic mixed refrigerant
A non-azeotropic mixing and refrigeration system technology, applied in refrigerators, refrigeration components, refrigeration and liquefaction, etc., can solve problems such as excessive cooling, and achieve the effect of improving the coefficient of performance
- Summary
- Abstract
- Description
- Claims
- Application Information
AI Technical Summary
Problems solved by technology
Method used
Image
Examples
Embodiment Construction
[0039] Hereinafter, embodiments will be described with reference to the accompanying drawings. Embodiments are not limited to the embodiments discussed below, and those skilled in the art who understand the spirit thereof will be able to easily come up with other embodiments falling within the scope by adding, modifying, and deleting components. However, this also falls into its spirit.
[0040] First, a preferably applicable zeotropic mixed refrigerant is proposed. In the description related to the selection of the non-azeotropic mixed refrigerant, the content of the present disclosure is divided into technical elements and described in detail. First, the process of selecting the type of zeotropic mixed refrigerant will be described.
[0041] Selection of the type of zeotropic refrigerant mixture
[0042] Refrigerants to be mixed suitable for non-azeotropic mixed refrigerants are proposed. As the refrigerant to be mixed, a hydrocarbon-based (HC-based) refrigerant can be s...
PUM
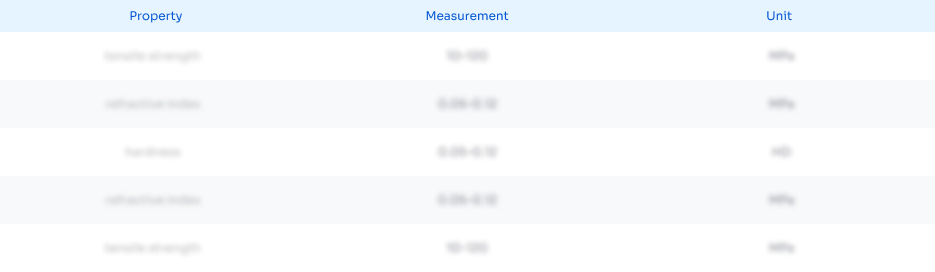
Abstract
Description
Claims
Application Information
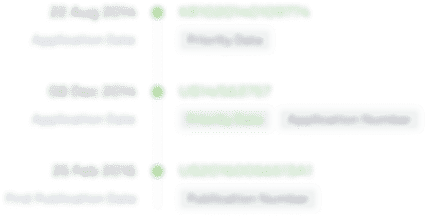
- Generate Ideas
- Intellectual Property
- Life Sciences
- Materials
- Tech Scout
- Unparalleled Data Quality
- Higher Quality Content
- 60% Fewer Hallucinations
Browse by: Latest US Patents, China's latest patents, Technical Efficacy Thesaurus, Application Domain, Technology Topic, Popular Technical Reports.
© 2025 PatSnap. All rights reserved.Legal|Privacy policy|Modern Slavery Act Transparency Statement|Sitemap|About US| Contact US: help@patsnap.com