Multi-energy park day-ahead economic dispatching method by considering demand response and containing electric vehicles
An electric vehicle, demand response technology, applied in electric vehicle charging technology, energy storage, AC network voltage adjustment and other directions, can solve the problem of new energy consumption that has not been solved.
- Summary
- Abstract
- Description
- Claims
- Application Information
AI Technical Summary
Problems solved by technology
Method used
Image
Examples
Embodiment Construction
[0143] In order to describe the technical solutions disclosed in the present invention in detail, the present invention will be further described below in conjunction with the accompanying drawings and specific embodiments.
[0144] The invention discloses a day-ahead economic scheduling method of a multi-energy park including electric vehicles considering demand response. The specific composition of the multi-energy park is as follows: figure 1 shown. The specific implementation steps are as follows: figure 2 Shown, technical scheme of the present invention comprises the following steps:
[0145] Step 1: Determine the specific composition of the multi-energy park, including the introduction of new energy forms and specific equipment composition;
[0146] The multi-energy park contains three energy types: electricity, gas and heat, so there are:
[0147] (1.1) The forms of new energy connected to the multi-energy park are: wind power and photovoltaic power generation;
...
PUM
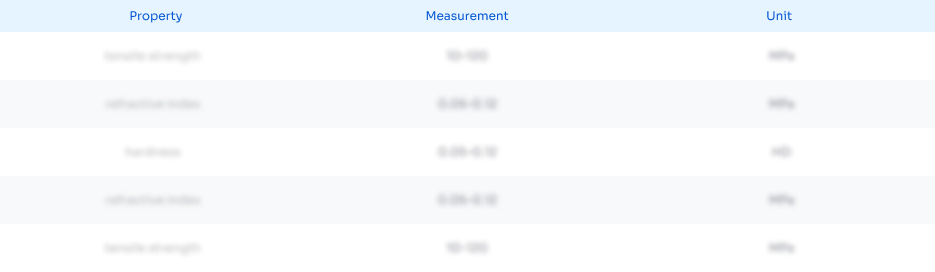
Abstract
Description
Claims
Application Information
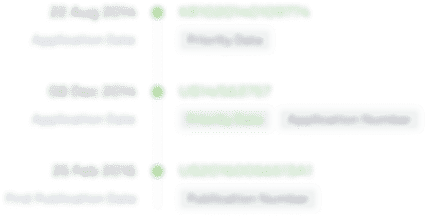
- R&D Engineer
- R&D Manager
- IP Professional
- Industry Leading Data Capabilities
- Powerful AI technology
- Patent DNA Extraction
Browse by: Latest US Patents, China's latest patents, Technical Efficacy Thesaurus, Application Domain, Technology Topic, Popular Technical Reports.
© 2024 PatSnap. All rights reserved.Legal|Privacy policy|Modern Slavery Act Transparency Statement|Sitemap|About US| Contact US: help@patsnap.com