Thermal diffuser and cooling apparatus for cooling heat source using the same
- Summary
- Abstract
- Description
- Claims
- Application Information
AI Technical Summary
Benefits of technology
Problems solved by technology
Method used
Image
Examples
first embodiment
[0028]A cooling apparatus 100A for cooling a heat source in the first embodiment will be described blow with reference to FIG. 1 and FIGS. 2A and 2B. FIG. 1 is an exploded perspective view illustrating the cooling apparatus 100A of the heat source, and FIGS. 2A and 2B are perspective views each illustrating a thermal diffuser 110 made of thermal conductivity plates in FIG. 1.
[0029]As shown in FIG. 1, the cooling apparatus 100A for cooling the heat source (hereinafter, simply referred to as the cooling apparatus 100A) includes the thermal diffuser 110, a heat source 120, an insulating plate 130, and a cooling unit 140. The cooling apparatus 100A cools the heat source 120 by transmitting heat of the heat source 120 to the cooling unit 140 through the thermal diffuser 110.
[0030]As shown in FIG. 2, the thermal diffuser 110 is a plate that efficiently transmits heat of the heat source 120 toward the cooling unit 140, and the thermal diffuser 110 includes multiple thermally-conductive pla...
second embodiment
[0038]FIGS. 3A to 3D show a thermal diffuser 110 of the second embodiment, and more specifically show a manufacturing process of the thermal diffuser 110. The method of manufacturing the thermal diffuser 110 in the second embodiment is different from the manufacturing method in the first embodiment (FIG. 1, FIG. 2).
[0039]The method for manufacturing the thermal diffuser 110 will be described below. Firstly, the plate-shaped thermally-conductive plates 111 having a better thermal conductivity in the plane direction than the thermal conductivity in the plate-thickness direction are prepared (FIG. 3A). The thermally-conductive plates 111 are laminated on one another in the plate-thickness direction to form a laminated body (FIG. 3B).
[0040]Next, the laminated body formed as above is cut in the lamination direction along the one side 111b of the thermally-conductive plate 111 to form a plate member as shown in FIG. 3C. Then, in the plate member, the plate surface 110a of the thermal diff...
third embodiment
[0042]FIGS. 4 to 6 show a cooling apparatus 100B of the third embodiment. In contrast to the first embodiment (FIGS. 1 and 2), the thermal diffuser 110 of the third embodiment is made of multiple thermal diffusers 110A, 110B.
[0043]As shown in FIGS. 4, 5A, and 5B, the thermal diffuser 110 is made of two thermal diffusers (or two laminated bodies). More specifically, the thermal diffuser 110 includes a first thermal diffuser 110A and a second thermal diffuser 1108. Each of the thermal diffusers 110A, 110B is similar to the thermal diffuser 110 described in the first embodiment. The thermal diffuser 110A is arranged such that the lamination direction of the thermally-conductive plates 111 of the thermal diffuser 110A is different from the lamination direction of the thermally-conductive plates 111 of the thermal diffuser 110B. Thus, the adjacent thermal diffusers (laminated bodies) 110A, 110B have the respective lamination directions of the thermally-conductive plates 111 different fro...
PUM
Property | Measurement | Unit |
---|---|---|
Angle | aaaaa | aaaaa |
Thickness | aaaaa | aaaaa |
Electrical conductor | aaaaa | aaaaa |
Abstract
Description
Claims
Application Information
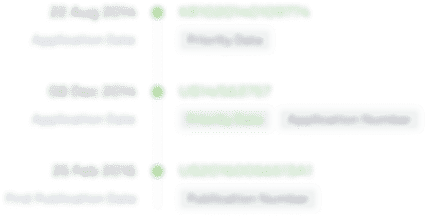
- Generate Ideas
- Intellectual Property
- Life Sciences
- Materials
- Tech Scout
- Unparalleled Data Quality
- Higher Quality Content
- 60% Fewer Hallucinations
Browse by: Latest US Patents, China's latest patents, Technical Efficacy Thesaurus, Application Domain, Technology Topic, Popular Technical Reports.
© 2025 PatSnap. All rights reserved.Legal|Privacy policy|Modern Slavery Act Transparency Statement|Sitemap|About US| Contact US: help@patsnap.com