Method and apparatus for controlling the common mode impedance misbalance of an isolated sigle-ended circuit
A common-mode impedance and circuit technology, applied in the direction of amplifier input/output impedance improvement, amplifiers with semiconductor devices/discharge tubes, electrical components, etc., can solve common-mode current reduction, common-mode current imbalance, and common-mode impedance increase And other issues
- Summary
- Abstract
- Description
- Claims
- Application Information
AI Technical Summary
Problems solved by technology
Method used
Image
Examples
Embodiment approach
[0045] Figure 2A with 2B The working principle of the described embodiment is to match the discrete capacitor with the parasitic capacitance formed between the shield and the ground layer or with the internal shield. in Figure 2A In the schematic diagram 34, a physical “outer” shield 42 enclosing an “inner” physical shield 46A is provided, and the “inner” physical shield 46A itself encloses the amplifier 26. One end of the noise source 18 is coupled to a capacitor 15 representing the parasitic capacitance between the ground ground and the noise source. The other end of the noise source 18 is coupled in parallel at terminal A to one end of a resistive wire (represented by resistor 22) and one end of another resistive wire (represented by resistor 24). The other end of resistor 22 is coupled to the input of amplifier 26, and the other end of resistor 24 is coupled to the reference of amplifier 26, which is also circuit grounded. In addition, a small signal source (not shown) inse...
PUM
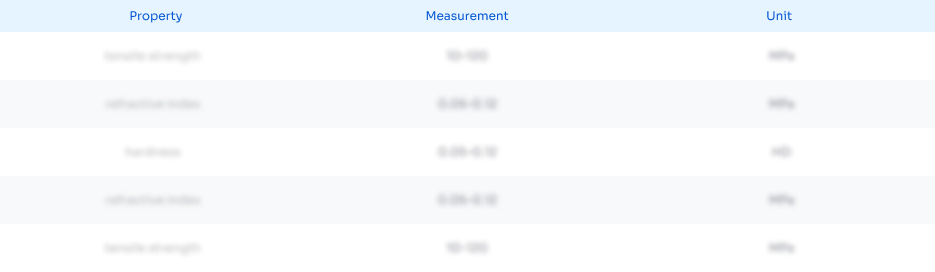
Abstract
Description
Claims
Application Information
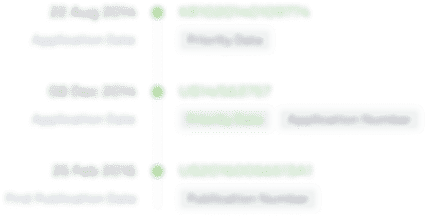
- R&D Engineer
- R&D Manager
- IP Professional
- Industry Leading Data Capabilities
- Powerful AI technology
- Patent DNA Extraction
Browse by: Latest US Patents, China's latest patents, Technical Efficacy Thesaurus, Application Domain, Technology Topic, Popular Technical Reports.
© 2024 PatSnap. All rights reserved.Legal|Privacy policy|Modern Slavery Act Transparency Statement|Sitemap|About US| Contact US: help@patsnap.com