Method of measuring an alignment mark or an alignment mark assembly, alignment system, and lithographic tool
A technology of aligning marks and alignment systems, which is applied in the photoengraving process, optics, instruments and other directions of the pattern surface, and can solve the problems of sensitivity and so on.
- Summary
- Abstract
- Description
- Claims
- Application Information
AI Technical Summary
Problems solved by technology
Method used
Image
Examples
Embodiment Construction
[0067] In this document, the terms "radiation" and "beam" are used to cover all types of electromagnetic radiation, including ultraviolet radiation (e.g. at wavelengths 365, 248, 193, 157 or 126 nm) and EUV (extreme ultraviolet radiation, e.g. in the range of about 5 to 100 nm).
[0068] The terms "reticle", "mask" or "patterning device" as employed herein may be broadly interpreted to refer to a general patterning device which may be used to impart a patterned cross-section to an incoming radiation beam , corresponding to the pattern to be created in the target portion of the substrate. In this context, the term "light valve" may also be used. Besides classical masks (transmissive or reflective, binary, phase-shifted, hybrid, etc.), other examples of such patterning devices include programmable mirror arrays and programmable LCD arrays.
[0069] figure 1 A lithographic apparatus LA is schematically depicted. The lithographic apparatus LA comprises an illumination system ...
PUM
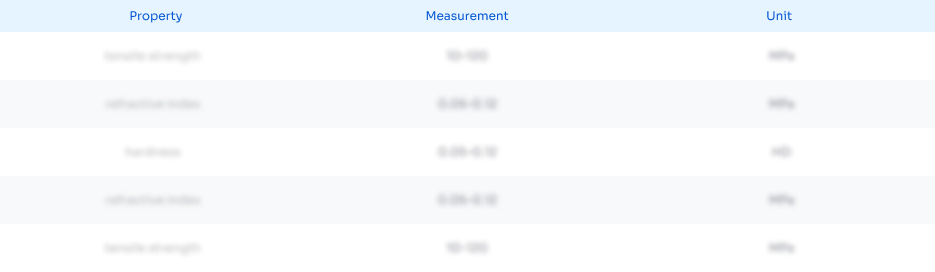
Abstract
Description
Claims
Application Information
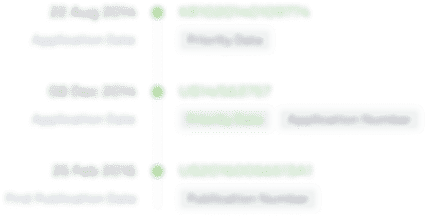
- R&D
- Intellectual Property
- Life Sciences
- Materials
- Tech Scout
- Unparalleled Data Quality
- Higher Quality Content
- 60% Fewer Hallucinations
Browse by: Latest US Patents, China's latest patents, Technical Efficacy Thesaurus, Application Domain, Technology Topic, Popular Technical Reports.
© 2025 PatSnap. All rights reserved.Legal|Privacy policy|Modern Slavery Act Transparency Statement|Sitemap|About US| Contact US: help@patsnap.com