Method for enhancing light-emitting efficiency of semiconductor type carbon nano-tube
A technology of carbon nanotubes and luminous efficiency, applied in semiconductor devices, electrical components, circuits, etc., can solve problems such as the luminous efficiency that cannot be achieved through carbon nanotubes
- Summary
- Abstract
- Description
- Claims
- Application Information
AI Technical Summary
Problems solved by technology
Method used
Image
Examples
Embodiment Construction
[0019] A method for improving the luminous efficiency of semiconducting carbon nanotubes, the method comprising:
[0020] Prepare carbon nanotube bundles containing semiconducting carbon nanotubes with different band gaps; the carbon nanotube bundles are all composed of semiconducting carbon nanotubes, or contain a small amount of metallic carbon nanotubes;
[0021] Wherein the method for preparing carbon nanotube bundles comprises:
[0022] a) Combining different carbon nanotubes into carbon nanotube bundles by using micromachines;
[0023] b) direct growth of carbon nanotube bundles using physical and chemical methods;
[0024] c) Dissolving carbon nanotubes into chemical reagents, separating and obtaining carbon nanotube bundles of different sizes by chemical and physical methods
[0025] The carbon nanotube bundle is prepared into a light-emitting device, and the excitation light that is close to or equal to the band gap of the wide bandgap semiconductor carbon nanotube ...
PUM
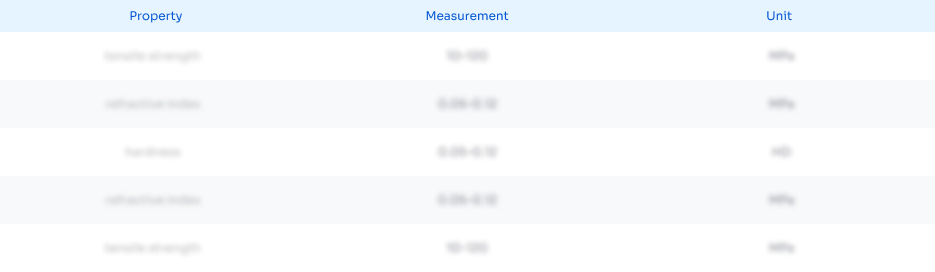
Abstract
Description
Claims
Application Information
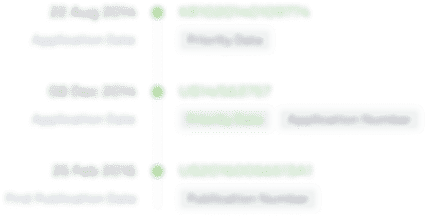
- Generate Ideas
- Intellectual Property
- Life Sciences
- Materials
- Tech Scout
- Unparalleled Data Quality
- Higher Quality Content
- 60% Fewer Hallucinations
Browse by: Latest US Patents, China's latest patents, Technical Efficacy Thesaurus, Application Domain, Technology Topic, Popular Technical Reports.
© 2025 PatSnap. All rights reserved.Legal|Privacy policy|Modern Slavery Act Transparency Statement|Sitemap|About US| Contact US: help@patsnap.com