Nanoscopic wire-based devices and arrays
a wire-based device and array technology, applied in nanoelectromechanical switches, instruments, relays, etc., can solve the problems of preventing current designs from functioning reliably, and reducing the size limitation of silicon-based microelectronics, so as to achieve the fundamental physical limitations of both device elements and wire interconnects, and preventing current designs from being made small
- Summary
- Abstract
- Description
- Claims
- Application Information
AI Technical Summary
Benefits of technology
Problems solved by technology
Method used
Image
Examples
example 1
[0093] To quantify the bistability and switching behavior of the device element a total energy, ET, can be calculated:
ET=Evdw+Eelas+Eelec (1)
where Evdw is the van der Waals (vdW) energy, Eelas is the elastic energy and Eelec is the electrostatic energy for the device. The first two terms in (1), which define the static potential, can be evaluated to assess the range of parameters that yield bistable devices. FIG. 11 shows plots of energy, ET=EvdW+Eelas, for a single 20 nm device as a function of separation at the junction. The series of curves correspond to initial separations of 0.8, 1.0, 1.2, 1.4, 1.6, 1.8, 2.0, 2.2, 2.4 nm for carbon nanotubes, with two well-defined minima observed for initial separations of 1.0 to 2.0 nm. These minima correspond to the crossing nanotubes being separated (2.4 nm) and in vdW contact (0.8 nm). The vdW interaction between nanotubes can be calculated by pairwise summation of a Lennard-Jones potential that has been shown previously to provide goo...
example 2
[0095] The effectiveness of switching the suspended nanotube devices between on and off states has been assessed by evaluating the voltage-dependent contribution of the electrostatic energy to the total energy. In this calculation, the boundary element method was used to numerically solve the Laplace equation for the complex three-dimensional geometry of the crossed nanotube device. Calculations of ET for switching a 20 nm device on and off (FIG. 15) demonstrate that it is possible to change reversibly between the on / off states using moderate voltages, which do not exceed the threshold field for nanotube failure. The switching voltages vary depending on the specific device geometry (i.e., shape of the static potential), and thus can be further optimized. For example, by using a thinner dielectric layer (that is, 4 vs 20 nm SiO2) the on and off switching thresholds can be reduced from 4.5 and 20 V to 3 and 5 V, respectively. The calculations also show that the electrostatic forces be...
example 3
[0097] Administration of reversible switching and the ability of the device to function as a non-volatile RAM is provided in this Example. Properties of suspended, crossed nanotube devices made from SWNT ropes were studied by mechanical manipulation (FIG. 16). Current-voltage (I-V) measurements made on the lower and upper nanotubes of a typical model device show ohmic behavior with resistances of 11 and 58 kΩ, respectively (FIG. 16A). The I-V curves between the upper and lower ropes in the off state were nonlinear, which is consistent with tunneling, with a resistance on the order of a GΩ. After switching on, the I-V curves exhibited ohmic behavior with a resistance of 112 kΩ (FIG. 16B). This large change in resistance is consistent with our predictions for off vs. on states in the suspended device architecture. Reversible switching between well-defined on / off states has also been observed in devices (FIG. 17). The smaller change in on / off resistances for the device in FIG. 17 is be...
PUM
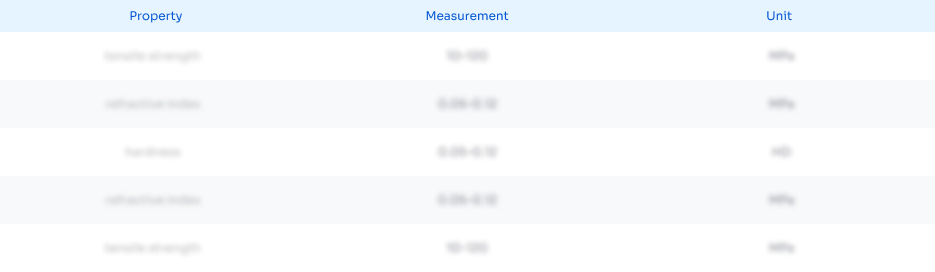
Abstract
Description
Claims
Application Information
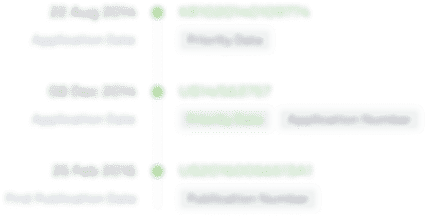
- R&D
- Intellectual Property
- Life Sciences
- Materials
- Tech Scout
- Unparalleled Data Quality
- Higher Quality Content
- 60% Fewer Hallucinations
Browse by: Latest US Patents, China's latest patents, Technical Efficacy Thesaurus, Application Domain, Technology Topic, Popular Technical Reports.
© 2025 PatSnap. All rights reserved.Legal|Privacy policy|Modern Slavery Act Transparency Statement|Sitemap|About US| Contact US: help@patsnap.com