Advanced Control Circuit for Switched-Mode DC-DC Converter
- Summary
- Abstract
- Description
- Claims
- Application Information
AI Technical Summary
Benefits of technology
Problems solved by technology
Method used
Image
Examples
Embodiment Construction
[0023]The preferred embodiments of the present invention provide significant advantages over DC-DC voltage converters of the prior art as will become evident from the following detailed description.
[0024]Referring to FIG. 4, there is a DC-DC voltage regulator circuit of the present invention. Here and in the following discussion, the same reference numerals are used in various drawing figures to indicate the same circuit elements. The voltage regulator includes a pulse width modulation (PWM) control circuit 400 that produces control signal PWM and complementary control signal / PWM. In some embodiments of the present invention, complementary control signal / PWM may be omitted. N-channel transistor 402 has a current path coupled between supply voltage VDD and voltage terminal VSW controlled by PWM. N-channel transistor 404 has a current path coupled between voltage terminal VSW and reference supply voltage terminal VSS. The current path of n-channel transistor 404 is controlled by / PW...
PUM
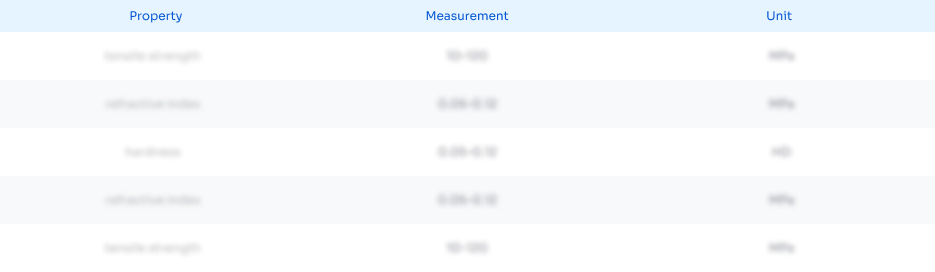
Abstract
Description
Claims
Application Information
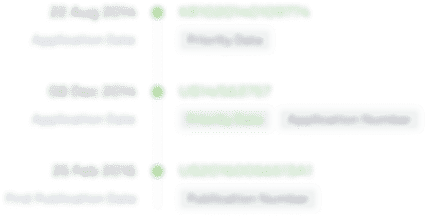
- Generate Ideas
- Intellectual Property
- Life Sciences
- Materials
- Tech Scout
- Unparalleled Data Quality
- Higher Quality Content
- 60% Fewer Hallucinations
Browse by: Latest US Patents, China's latest patents, Technical Efficacy Thesaurus, Application Domain, Technology Topic, Popular Technical Reports.
© 2025 PatSnap. All rights reserved.Legal|Privacy policy|Modern Slavery Act Transparency Statement|Sitemap|About US| Contact US: help@patsnap.com