Method and system for synchronizing time and frequency in orthogonal frequency division multiplex communication
A frequency synchronization and multiplexing communication technology, applied in the direction of multi-frequency code system, etc., can solve the problems of data detection interference, reduce system overhead, algorithm performance degradation, etc., achieve low overhead, achieve the effect of time and frequency synchronization
- Summary
- Abstract
- Description
- Claims
- Application Information
AI Technical Summary
Problems solved by technology
Method used
Image
Examples
Embodiment Construction
[0075] The method for time and frequency synchronization between the transmitted signal and the received signal in OFDM communication provided by the embodiment of the present invention can be used in a MIMO system. The method is a joint process including the construction of the transmitted data at the transmitting end and the time and frequency synchronization at the receiving end using the specially structured transmitted data. The idea is to use pilots in the communication process. By designing the frequency-domain joint pilot patterns of each antenna at the transmitting end, the time-domain sequences have specific correlations, and then use the time-domain correlations to synchronize time and frequency.
[0076] The MIMO-OFDM time and frequency synchronization method and system provided by the embodiments of the present invention will be described in detail below with reference to the accompanying drawings.
[0077] In a preferred embodiment of the present invention, a sch...
PUM
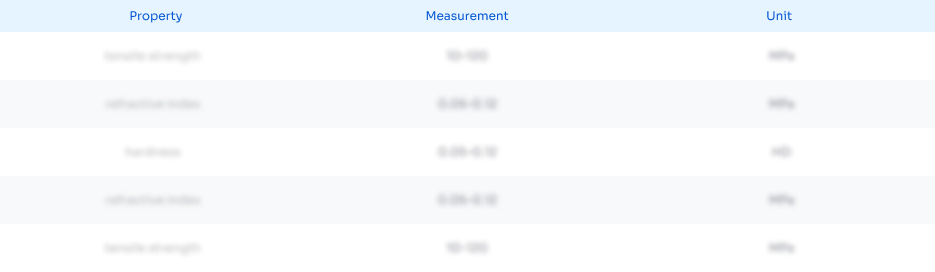
Abstract
Description
Claims
Application Information
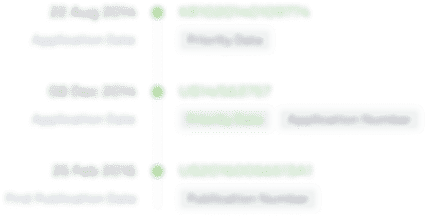
- R&D Engineer
- R&D Manager
- IP Professional
- Industry Leading Data Capabilities
- Powerful AI technology
- Patent DNA Extraction
Browse by: Latest US Patents, China's latest patents, Technical Efficacy Thesaurus, Application Domain, Technology Topic, Popular Technical Reports.
© 2024 PatSnap. All rights reserved.Legal|Privacy policy|Modern Slavery Act Transparency Statement|Sitemap|About US| Contact US: help@patsnap.com