Semiconductor photoelectric structure for improving light extraction efficiency and manufacturing method thereof
An extraction efficiency, semiconductor technology, applied in semiconductor devices, circuits, electrical components, etc., to improve light extraction efficiency, reduce defect density, and improve epitaxy quality.
- Summary
- Abstract
- Description
- Claims
- Application Information
AI Technical Summary
Problems solved by technology
Method used
Image
Examples
Embodiment Construction
[0073] The direction of the present invention discussed here is a semiconductor optoelectronic element structure and its manufacturing method which can improve the light extraction efficiency. In order to provide a thorough understanding of the present invention, detailed steps and components thereof will be set forth in the following description. Obviously, the practice of the invention is not limited to specific details known to those skilled in the semiconductor optoelectronic process. On the other hand, well-known components or steps have not been described in detail so as not to unnecessarily limit the invention. The preferred embodiments of the present invention will be described in detail as follows, but in addition to these detailed descriptions, the present invention can also be widely implemented in other embodiments, and the scope of the present invention is not limited, it is based on the following claims .
[0074] An object of the present invention is to improv...
PUM
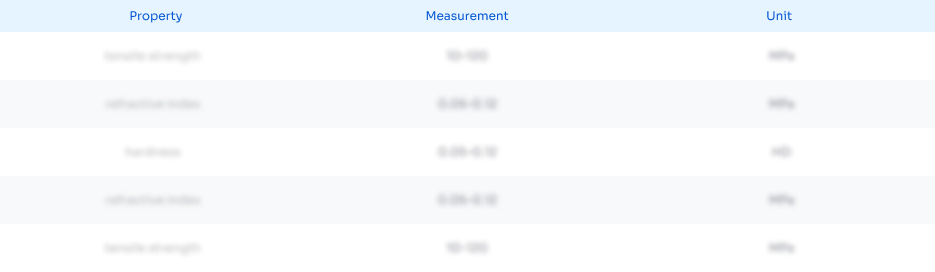
Abstract
Description
Claims
Application Information
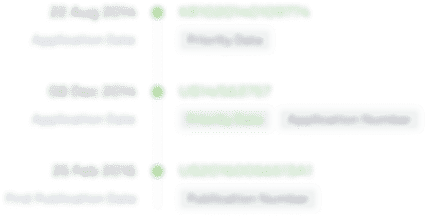
- Generate Ideas
- Intellectual Property
- Life Sciences
- Materials
- Tech Scout
- Unparalleled Data Quality
- Higher Quality Content
- 60% Fewer Hallucinations
Browse by: Latest US Patents, China's latest patents, Technical Efficacy Thesaurus, Application Domain, Technology Topic, Popular Technical Reports.
© 2025 PatSnap. All rights reserved.Legal|Privacy policy|Modern Slavery Act Transparency Statement|Sitemap|About US| Contact US: help@patsnap.com