Method of preventing adverse effects by glp-1
A technology of GLP-1 and preparations, which can be used in pharmaceutical formulas, medical preparations containing active ingredients, metabolic diseases, etc., and can solve problems such as toxicity to patients
- Summary
- Abstract
- Description
- Claims
- Application Information
AI Technical Summary
Problems solved by technology
Method used
Image
Examples
Embodiment 1
[0116] Administration of GLP-1 to healthy adult males in the form of an inhalable dry powder
[0117] GLP-1 has been shown to control elevated blood glucose in humans when given by intravenous (iv) or subcutaneous (sc) infusion or by multiple subcutaneous injections. Due to the extremely short half-life of the hormone, continuous subcutaneous infusions or multiple daily subcutaneous injections are required to achieve clinical efficacy. None of these routes is practical for prolonged clinical use. The applicant has found in animal experiments that therapeutic levels can be achieved when GLP-1 is administered by inhalation. The results of these studies can be found, for example, in US Patent Application No. 11 / 735,957, the disclosure of which is incorporated herein by reference.
[0118] In healthy individuals, several effects of GLP-1, including decreased gastric emptying, increased satiety and suppression of inappropriate glucagon secretion, appear to be related to the bur...
Embodiment 2
[0147] Comparison of pulmonary administration of GLP-1 and Exented to male Zucker diabetic obese rats for and subcutaneous administration of Exented
[0148] Considerable effort has been expended in developing GLP-1 analogs with longer circulating half-lives that would lead to clinically useful treatments. As demonstrated herein, pulmonary administration of GLP-1 also provided clinically meaningful activity. It is therefore of interest to compare these two pathways.
[0149] Preparation of FDKP particles
[0150] Fumaryl diketopiperazine (FDKP) and polysorbate 80 were dissolved in dilute ammonia water to obtain a solution containing 2.5 wt% FDKP and 0.05 wt% polysorbate 80. The FDKP solution was then mixed with an acetic acid solution containing polysorbate 80 to form granules. The particles were washed and concentrated by tangential flow filtration to achieve about 11% solids by weight.
[0151] Preparation of GLP-1 stock solution .
[0152] A 10 wt% GLP-1 stock ...
Embodiment 3
[0166] Oxyntomodulin / FDKP Powder Preparation
[0167] Oxyntomodulin (also known as glucagon-37) is a peptide consisting of 37 amino acid residues. This peptide is manufactured by and obtained from American Peptide Company, Inc. of Sunnyvale, CA. FDKP particles in suspension were mixed with oxyntomodulin solution, then snap-frozen into pellets in liquid nitrogen, and lyophilized to produce sample powders.
[0168] Six powders were prepared with target peptide contents between 5% and 30%. The actual peptide content determined by HPLC was between 4.4% and 28.5%. The aerodynamic properties of powders containing 10% peptide were analyzed using cascade impact.
[0169] The FDKP solution was then mixed with a polysorbate 80-containing acetic acid solution to form granules. The particles were washed and concentrated by tangential flow filtration to achieve about 11% solids by weight.
[0170] The FDKP particle suspension (1885 mg x 11.14% solids = 210 mg FDKP particles) was weigh...
PUM
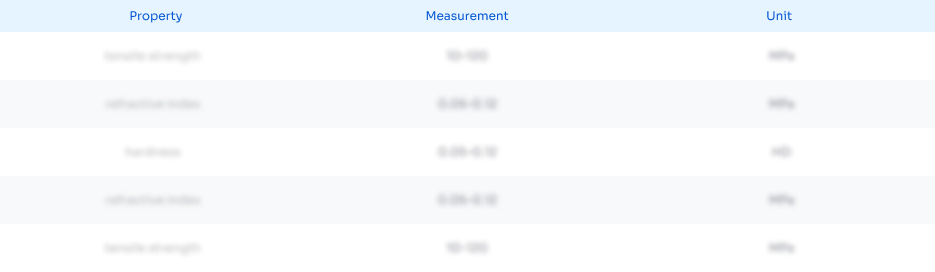
Abstract
Description
Claims
Application Information
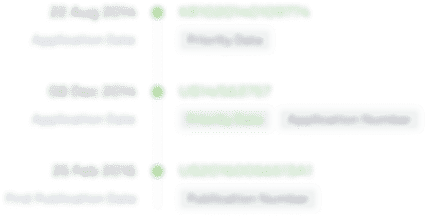
- R&D Engineer
- R&D Manager
- IP Professional
- Industry Leading Data Capabilities
- Powerful AI technology
- Patent DNA Extraction
Browse by: Latest US Patents, China's latest patents, Technical Efficacy Thesaurus, Application Domain, Technology Topic.
© 2024 PatSnap. All rights reserved.Legal|Privacy policy|Modern Slavery Act Transparency Statement|Sitemap