Y-branch-based thermo-optic digital optical switches and variable optical attenuators with non-uniform heating
a digital optical switch and variable optical technology, applied in the field of optical communication technology, can solve the problems of limiting the applicability of integrated optical circuits, difficult control of such devices, and easy error prone manufacturing
- Summary
- Abstract
- Description
- Claims
- Application Information
AI Technical Summary
Benefits of technology
Problems solved by technology
Method used
Image
Examples
example 1
[0054] In this Example, the following terms are employed:
[0055] The composition designated B3 was prepared by combining 94% by weight of ethoxylated perfluoropolyether diacrylate (MW1100), 4% by weight of di-trimethylolpropane tetraacrylate, and 2% by weight of Darocur 1173, a photoinitiator available from Ciba-Geigy.
[0056] The composition designated BF3 was prepared by combining 98% by weight of ethoxylated perfluoropolyether diacrylate (MW1100) and 2% by weight of Darocur 1173.
[0057] The composition designated C3 was prepared by combining 91% by weight of ethoxylated perfluoropolyether diacrylate (MW1100), 6.5% by weight of di-trimethylolpropane tetra-acrylate, 2% by weight of Darocur1173, and 0.5% by weight of Darocur 4265 a different photoinitiator available from Ciba Geigy.
[0058] The following fabrication process was performed twice, once with mask designated P03, once with mask designated P05 (see FIG. 2 for structures of each mask).
example 2
[0062] A second Y-branch specimen, prepared in a manner identical to that in Example 1, was evaluated according to the following protocol: The measurement was done in 2 steps:
[0063] 1. 1.55 micrometer wavelength light was coupled from a glass optical fiber input into the Y-branch trunk and after traversing the device the light was couple to a glass optical fiber at the output of the ‘right’ branch and was sent to a photodetector. Electrical power was applied to the left branch heater and was changed continuously from 50 mW to 0 mW, then electrical power was applied to the right heater and was changed continuously from 0 mW to 50 mW. The optical power attenuation measured at the photodetector is indicated by the blue line in FIG. 6.
[0064] 2. Light was launched as in the preceding paragraph. but was coupled to a photodetector at the output of the ‘left’ branch Electrical power was applied to the right heater and was changed continuously from 50 mW to 0 mW, then electrical power was ...
PUM
Property | Measurement | Unit |
---|---|---|
angle | aaaaa | aaaaa |
angle | aaaaa | aaaaa |
distance | aaaaa | aaaaa |
Abstract
Description
Claims
Application Information
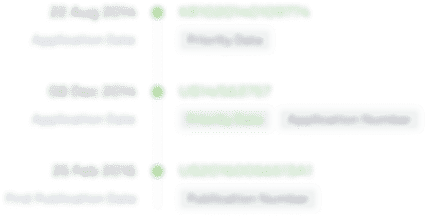
- R&D Engineer
- R&D Manager
- IP Professional
- Industry Leading Data Capabilities
- Powerful AI technology
- Patent DNA Extraction
Browse by: Latest US Patents, China's latest patents, Technical Efficacy Thesaurus, Application Domain, Technology Topic, Popular Technical Reports.
© 2024 PatSnap. All rights reserved.Legal|Privacy policy|Modern Slavery Act Transparency Statement|Sitemap|About US| Contact US: help@patsnap.com