Photocatalytic water splitting
A technology of photocatalysis and photocatalysis, applied in the direction of physical/chemical process catalyst, inorganic chemistry, electrolysis process, etc., can solve problems such as difficult separation and not very effective
- Summary
- Abstract
- Description
- Claims
- Application Information
AI Technical Summary
Problems solved by technology
Method used
Image
Examples
Embodiment Construction
[0026]In one embodiment, the thin conductive isolation layer is mechanically supported by one or more perforated supports. This is particularly advantageous when the electrically conductive isolating layer is so thin that it has low mechanical stability itself. The perforated carrier may be provided on one or both surfaces of the conductive isolation layer. Since the support is perforated, it enables the photocatalyst to directly contact the conductive isolation layer, which is of course desirable in terms of efficient electron transfer.
[0027] The perforated support has a thickness in the range of 50-1000 μm, preferably in the range of 100-750 μm, which can be determined by scanning and / or transmission electron microscopy. A suitable and practical carrier is, for example, a silicon carrier, such as a silicon wafer. If necessary, the carrier can be perforated, such as by a standard etching process (process, process). In many cases it is advantageous to produce an electric...
PUM
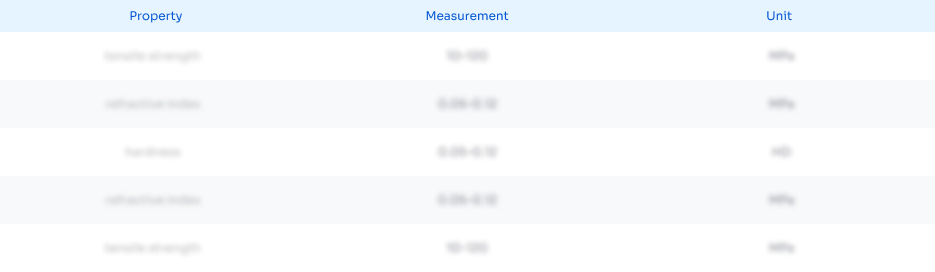
Abstract
Description
Claims
Application Information
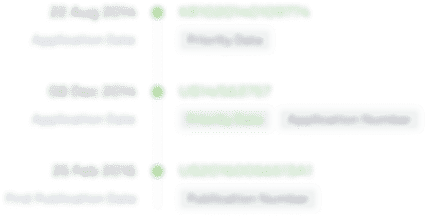
- Generate Ideas
- Intellectual Property
- Life Sciences
- Materials
- Tech Scout
- Unparalleled Data Quality
- Higher Quality Content
- 60% Fewer Hallucinations
Browse by: Latest US Patents, China's latest patents, Technical Efficacy Thesaurus, Application Domain, Technology Topic, Popular Technical Reports.
© 2025 PatSnap. All rights reserved.Legal|Privacy policy|Modern Slavery Act Transparency Statement|Sitemap|About US| Contact US: help@patsnap.com