Electron tube
A technology of electron tubes and electrons, applied in the field of electron tubes, can solve problems such as the reduction of the output of photomultiplier tubes and the deterioration of the sensitivity of the photoelectric surface
- Summary
- Abstract
- Description
- Claims
- Application Information
AI Technical Summary
Problems solved by technology
Method used
Image
Examples
Embodiment Construction
[0023] Next, preferred embodiments of the photomultiplier tube of the present invention will be described together with the drawings. In the description of the drawings, the same reference numerals are attached to the same elements, and overlapping descriptions are omitted. In addition, the dimensional ratios of the drawings do not necessarily match the components described.
[0024] figure 2 It is a cross-sectional view of a photomultiplier tube according to an embodiment of the present invention. The photomultiplier tube is formed by disposing the electron multiplier 24 composed of multistage dynode electrodes 24a to 24n inside the vacuum container 10. The vacuum container 10 is formed by the following parts: a circular light-receiving panel 11 that receives incident light; A cylindrical metal side pipe 12 and a circular stem 13 constituting a base portion are formed on the outer peripheral portion of the light receiving panel 11 .
[0025] A semiconductor photoelectric s...
PUM
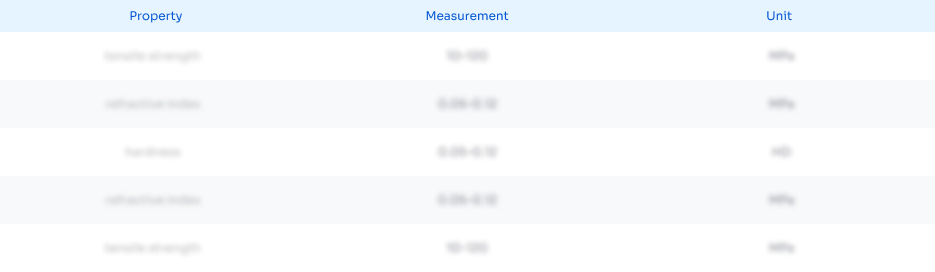
Abstract
Description
Claims
Application Information
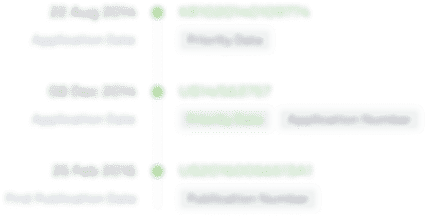
- Generate Ideas
- Intellectual Property
- Life Sciences
- Materials
- Tech Scout
- Unparalleled Data Quality
- Higher Quality Content
- 60% Fewer Hallucinations
Browse by: Latest US Patents, China's latest patents, Technical Efficacy Thesaurus, Application Domain, Technology Topic, Popular Technical Reports.
© 2025 PatSnap. All rights reserved.Legal|Privacy policy|Modern Slavery Act Transparency Statement|Sitemap|About US| Contact US: help@patsnap.com