Plasma processing apparatus
- Summary
- Abstract
- Description
- Claims
- Application Information
AI Technical Summary
Benefits of technology
Problems solved by technology
Method used
Image
Examples
Embodiment Construction
[0034]The embodiments of the present invention will be described with reference to the accompanying drawings which form a part hereof.
[0035]FIG. 1 shows a configuration of a plasma etching apparatus in accordance with a first embodiment of the present invention. The plasma etching apparatus is configured as a capacitively coupled plasma etching apparatus of cathode coupled type having parallel plate electrodes, and includes a cylindrical chamber (processing vessel) 10 made of a metal such as aluminum, stainless steel or the like. The chamber 10 is frame grounded.
[0036]A circular plate-shaped susceptor 12 serving as a lower electrode for mounting thereon a substrate to be processed, e.g., a semiconductor wafer W, is disposed horizontally in the chamber 10. The susceptor 12 is made of, e.g., aluminum, and is supported by a cylindrical insulating supporting portion 14 which is made of ceramic and vertically extends from a bottom of the chamber 10 without being grounded. An annular gas ...
PUM
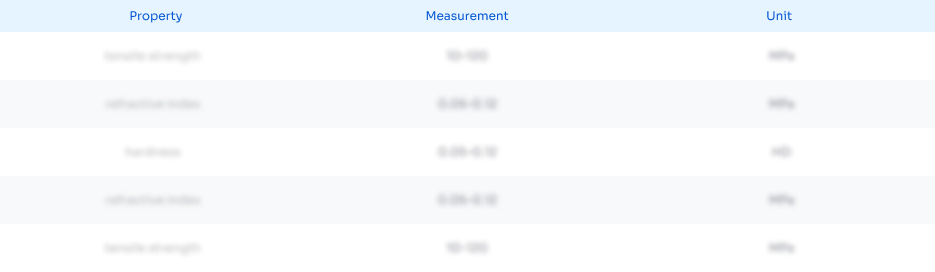
Abstract
Description
Claims
Application Information
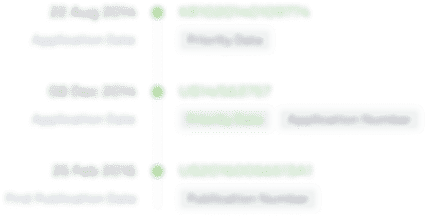
- Generate Ideas
- Intellectual Property
- Life Sciences
- Materials
- Tech Scout
- Unparalleled Data Quality
- Higher Quality Content
- 60% Fewer Hallucinations
Browse by: Latest US Patents, China's latest patents, Technical Efficacy Thesaurus, Application Domain, Technology Topic, Popular Technical Reports.
© 2025 PatSnap. All rights reserved.Legal|Privacy policy|Modern Slavery Act Transparency Statement|Sitemap|About US| Contact US: help@patsnap.com