Metal oxide semiconductor field effect transistor (MOSFET) with lifted silicide source drain contact and manufacture method thereof
A manufacturing method and a technology of source-drain contact, applied in semiconductor/solid-state device manufacturing, semiconductor devices, electrical components, etc., can solve the problems of reducing the total resistance of the device, failing to effectively reduce the interconnection resistance, and affecting the electrical performance of semiconductor devices. The effect of reducing interconnection resistance, reducing dosage, and improving electrical performance
- Summary
- Abstract
- Description
- Claims
- Application Information
AI Technical Summary
Problems solved by technology
Method used
Image
Examples
Embodiment Construction
[0029] The features and technical effects of the technical solution of the present invention will be described in detail below with reference to the accompanying drawings and in conjunction with exemplary embodiments, and a semiconductor device capable of effectively reducing interconnection resistance and a manufacturing method thereof are disclosed. It should be pointed out that similar reference numerals represent similar structures, and the terms "first", "second", "upper", "lower" and the like used in this application can be used to modify various device structures or manufacturing processes . These modifications do not imply spatial, sequential or hierarchical relationships of the modified device structures or fabrication processes unless specifically stated.
[0030] First, refer to image 3 , forming contact holes in the base structure.
[0031] Specifically, the substrate 10 is provided first. The substrate 10 may be bulk silicon, silicon-on-insulator (SOI) or a co...
PUM
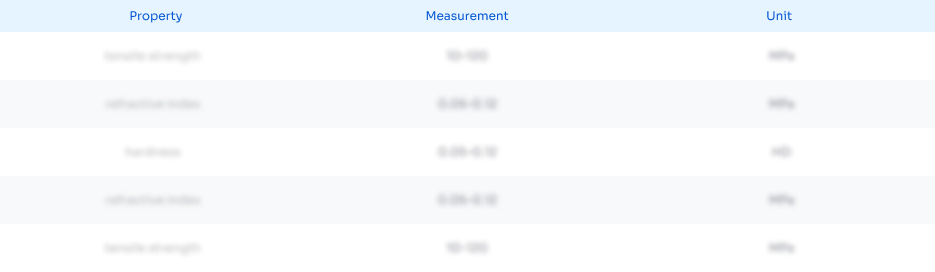
Abstract
Description
Claims
Application Information
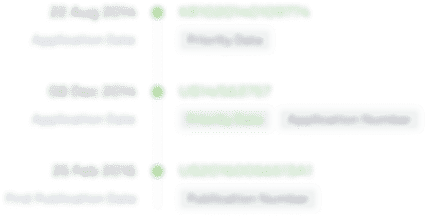
- R&D
- Intellectual Property
- Life Sciences
- Materials
- Tech Scout
- Unparalleled Data Quality
- Higher Quality Content
- 60% Fewer Hallucinations
Browse by: Latest US Patents, China's latest patents, Technical Efficacy Thesaurus, Application Domain, Technology Topic, Popular Technical Reports.
© 2025 PatSnap. All rights reserved.Legal|Privacy policy|Modern Slavery Act Transparency Statement|Sitemap|About US| Contact US: help@patsnap.com