Reflector and susceptor assembly for chemical vapor deposition reactor
A susceptor and reflector technology, applied in gaseous chemical plating, metal material coating process, coating and other directions, can solve problems such as reducing useful life
- Summary
- Abstract
- Description
- Claims
- Application Information
AI Technical Summary
Problems solved by technology
Method used
Image
Examples
Embodiment Construction
[0019] The present invention can be applied to a wide variety of possible reactors where uniformity of temperature rise of the substrate is important or highly desired, especially for producing uniform deposition of materials on such substrates. Although the examples given herein are CVD cold wall reactors in which the heater is in the form of an array of one or more wire lamps, other similar reactors are considered to be encompassed by the present invention. Improvements herein provide processing kits for radiatively heated susceptors in which one or more IR reflector elements compensate for non-uniform heating profiles from any type of heater employed by the reactor.
[0020] exist figure 1 In , a part of the cold wall reactor 11 supports the showerhead diffuser plate 101 and the showerhead assembly above the gas supply manifold 103 . One end of the reactor 11 can be seen on the right side of the figure, while the left hand side of the figure corresponds to the central part...
PUM
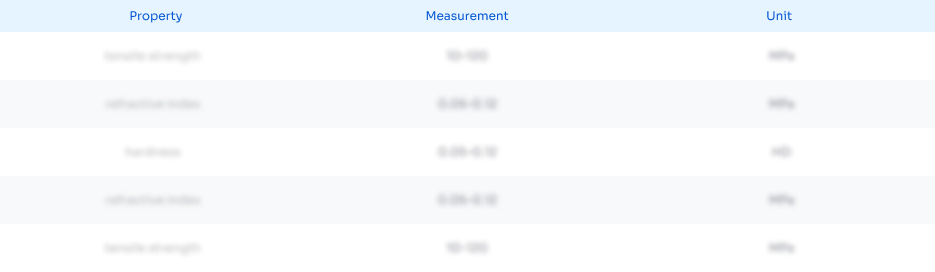
Abstract
Description
Claims
Application Information
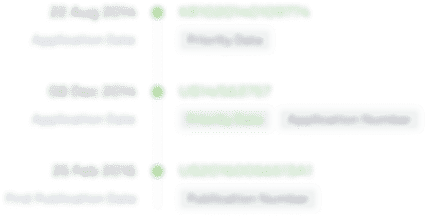
- Generate Ideas
- Intellectual Property
- Life Sciences
- Materials
- Tech Scout
- Unparalleled Data Quality
- Higher Quality Content
- 60% Fewer Hallucinations
Browse by: Latest US Patents, China's latest patents, Technical Efficacy Thesaurus, Application Domain, Technology Topic, Popular Technical Reports.
© 2025 PatSnap. All rights reserved.Legal|Privacy policy|Modern Slavery Act Transparency Statement|Sitemap|About US| Contact US: help@patsnap.com