Spin transfer torque magnetic memory device using magnetic resonance precession and the spin filtering effect
- Summary
- Abstract
- Description
- Claims
- Application Information
AI Technical Summary
Benefits of technology
Problems solved by technology
Method used
Image
Examples
embodiment
[0073]The effect of the magnetic memory device according to the present invention was confirmed through micro-magnetic modeling using an equation of motion of magnetization. The justification of this method was efficiently secured through a conventional computer hard disk development and spin-transfer-torque research.
[0074]The equation of motion of the magnetization is expressed by the following equation 3.
∂m1∂f=-γ(m1×H2eff)+αm1×∂m1∂t-γℏη1je2eMS1d1m1×(m1×p1)+γℏjeη2→12eMS1d1m1×(m1×m2)∂m2∂f=-γ(m2×H2eff)+αm2×∂m2∂t-γℏη2je2eMSd2m2×(m2×m1)+γℏη3je2eMS2d2m1×(m1×p2)p1=z^,p2=-z^[Equation3]
[0075]In the equation 3,
[0076]“m1” and “m2” denote unit magnetization vectors of the first free magnetic layer 203 and 303 and the second free magnetic layer 205 and 305, respectively, “γ” denotes a magnetic rotational constant, “H1eff” and “H2eff” denotes entire effective magnetic field vectors of the first free magnetic layer 203 and 303 and the second free magnetic layer 205 and 305, respectively, “α” den...
experimental example 1
Magnetization Behavior of First Free Magnetic Layer and Second Free Magnetic Layer According to a Time Caused by Applying a Current to the Device According to the Present Invention
[0077](1) There is illustrated magnetization behavior of the first free magnetic layer having the perpendicular anisotropy and the second free magnetic layer having the horizontal anisotropy when the current is applied to the magnetic memory device according to an embodiment of the present invention as shown in the following FIG. 2.
[0078](2) Structure and physical property values of the device are as follows:
[0079]sectional area of an entire structure=314 nm2,
[0080]fixed magnetic layer 201 / first non-magnetic layer 202 / first free magnetic layer 203: “thickness (t)=3 nm, perpendicular anisotropy constant (K⊥)=6×106 erg / cm3, saturation magnetization value (MS1)=1000 emu / cm3, Gilbert damping constant (α)=0.01, and spin polarization efficiency constant (η1)=1.0”,
[0081]second non-magnetic layer 204: thickness t=...
experimental example 2
Measurement of Switching Probabilities with Respect to Currents Applied to a Device According to the Present Invention and a Device According to a Conventional Structure
[0091](1) There are illustrated switching currents with respect to the conventional structure of FIG. 1 and the new structure according to the present invention of FIG. 2.
[0092](2) Structure and physical property values of the devices are as follows.
[0093]A sectional area of an entire structure of each of the two structures is 314 nm2.
[0094]The conventional structure of FIG. 1 has fixed magnetic layer 101 / non-magnetic layer 102 / free magnetic layer 103: “thickness (t)=3 nm, perpendicular anisotropy constant (K⊥)=6×106 erg / cm3, saturation magnetization value (MS1)=1000 emu / cm3, Gilbert damping constant (α)=0.01, and spin polarization efficiency constant (η1)=1.0”.
[0095]The physical property values of the new structure according to the present invention are as follows:
[0096]fixed magnetic layer 201 / first non-magnetic la...
PUM
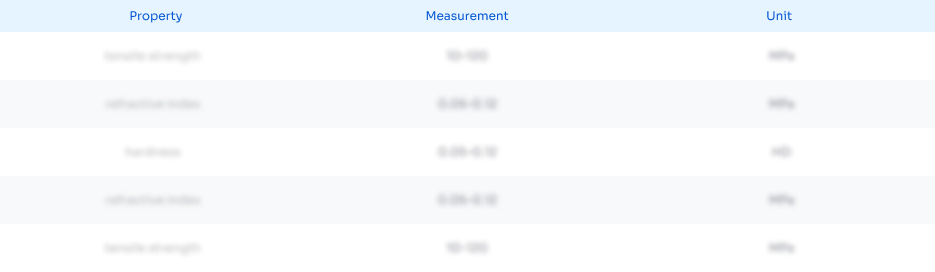
Abstract
Description
Claims
Application Information
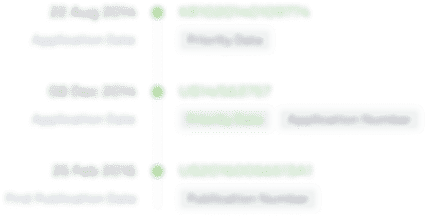
- R&D
- Intellectual Property
- Life Sciences
- Materials
- Tech Scout
- Unparalleled Data Quality
- Higher Quality Content
- 60% Fewer Hallucinations
Browse by: Latest US Patents, China's latest patents, Technical Efficacy Thesaurus, Application Domain, Technology Topic, Popular Technical Reports.
© 2025 PatSnap. All rights reserved.Legal|Privacy policy|Modern Slavery Act Transparency Statement|Sitemap|About US| Contact US: help@patsnap.com