Low bandgap, monolithic, multi-bandgap, optoelectronic devices
a low bandgap, monolithic technology, applied in the direction of semiconductor/solid-state device manufacturing, semiconductor/solid-state device manufacturing, electrical apparatus, etc., can solve the problems of reducing energy conversion efficiency, large inefficiencies and energy losses to unwanted heat, and knowledge alone cannot solve the problem of making an efficient and useful energy conversion devi
- Summary
- Abstract
- Description
- Claims
- Application Information
AI Technical Summary
Benefits of technology
Problems solved by technology
Method used
Image
Examples
embodiment 110
[0082] As mentioned above, the numbers and combinations of subcells and lattice constant transition layers as well as the specific example bandgap values shown in the PV converter 112 of FIG. 5 are selected arbitrarily to illustrate the principles of this invention. The only requirement is that the incident radiation reaches the subcells in order of decreasing bandgaps, so that the shorter wavelength radiation is absorbed and converted to electricity by higher bandgap subcells that will transmit unabsorbed, longer wavelength radiation to the next subcell(s). Other details, such as buffer layers, tunnel junction or isolation layers, contacts, optic control layers, etc., for fabricating a working PV converter can be similar to those described above for either the series connected subcell embodiments 10 of FIGS. 1 and 2 or the independently connected subcell embodiment 110 of FIG. 4.
embodiment 10
[0083] Now, as illustrated in another alternative inverted, monofacial, multi-bandgap, PV converter 140 in FIG. 6, the positions of the transparent lattice constant transition layer 20 and the first subcell 22 positions can be reversed from their positions shown in the FIG. 1 embodiment 10. Specifically, the lattice constant transition layer 20 can be grown expitaxially on the InP substrate 26 by gradually adding more and more As to the growing InAsyP1-y lattice constant transition layer 20, as described above, until a desired lattice constant is attained for a desired GaxIn1-xAs or GaxIn1-xAsyP1-y semiconductor material with a desired bandgap to be grown on the InP substrate 26. As explained above, the desire bandgap is chosen for absorbing and converting infrared radiation R of a desired wavelength or frequency band to electricity.
[0084] For example, but not for limitation, if it is desired to have the first subcell 22 in the PV converter 140 of FIG. 6 absorb and convert infrared ...
embodiment 140
[0089] This invention, as mentioned above, also extends to low bandgap, monolithic, multi-bandgap PV converters with more than one lattice constant transition layer. For example, referring again to FIG. 6, one or more additional subcells with even lower bandgap(s) than the 0.58 eV bandgap of the second subcell 24 can be grown on top of subcell 24. Such an example PV converter 150 with three subcells 22, 24, 72 is illustrated diagrammatically in FIG. 8. This example three-bandgap PV converter 150 is illustrated for convenience with the same substrate 26, first lattice constant transition layer 20, first subcell 22, and second subcell 24 as the two-bandgap embodiment 140 of FIG. 6, but it has a second lattice constant transition layer 70 positioned between the second subcell 24 and a third subcell 72.
[0090] As was explained above in relation to the inverted tandem (two-subcell) PV converter 140 in FIG. 6, the InP substrate 26 and the first lattice constant transition layer 20 are tran...
PUM
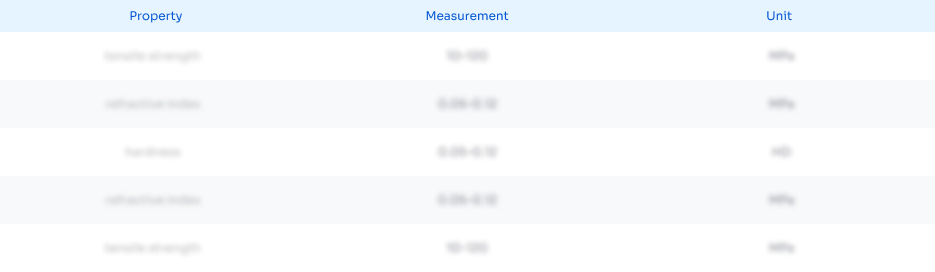
Abstract
Description
Claims
Application Information
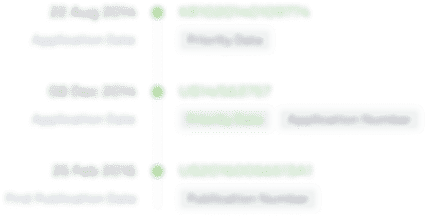
- R&D Engineer
- R&D Manager
- IP Professional
- Industry Leading Data Capabilities
- Powerful AI technology
- Patent DNA Extraction
Browse by: Latest US Patents, China's latest patents, Technical Efficacy Thesaurus, Application Domain, Technology Topic, Popular Technical Reports.
© 2024 PatSnap. All rights reserved.Legal|Privacy policy|Modern Slavery Act Transparency Statement|Sitemap|About US| Contact US: help@patsnap.com