Production of nickel nanoparticles from a nickel precursor via laser pyrolysis
a technology of laser pyrolysis and nickel nanoparticles, which is applied in the field of production of nickel nanoparticles, can solve problems such as detriment to magnetic properties, and achieve the effects of increasing co flow rate, increasing production rate first, and increasing flow ra
- Summary
- Abstract
- Description
- Claims
- Application Information
AI Technical Summary
Benefits of technology
Problems solved by technology
Method used
Image
Examples
example 1
Experimental Methods
[0043] The reactor configuration shown in FIGS. 1 and 2 was used to prepare nickel nanoparticles in the experiments described herein. Because nickel carbonyl is highly toxic, it was generated in situ by flowing CO through a tube packed with nickel powder, which was placed inside of a tube furnace. This safe and convenient method was applied to generate a small flow of nickel carbonyl to the reactor without maintaining any inventory of nickel carbonyl. Details of this nickel carbonyl generator were as follows. About 605 g of nickel powder (laboratory grade, reduced powder, Fisher Chemicals, Fairlawn, N.J.) was packed into a 1-inch o.d. stainless steel tube with a packed length of about 14 inches. Glass wool and a porous frit were packed at both ends to prevent the nickel powder from leaving the tube. This nickel powder was heated to 300-350° C. in a stream of flowing H2 (250 sccm) for at least 60 minutes to remove any oxide on the nickel surface. The tube was the...
example 2
Collection of Nickel Particles
[0046] The nickel particles collected on filters were loosely agglomerated and black in color. They were attracted to a permanent magnet, both as a powder and when dispersed in a solvent. They were readily dispersed in nonpolar solvents, such as toluene or hexane, and their dispersion was improved by the addition of oleic acid or oleyl amine. However, they did not form colloidal dispersions with long-term stability. Over a period of hours to days, depending on particle size, presence of surfactant, and particle preparation conditions, particles agglomerated and sedimented out of solution. This process was accelerated in the presence of a magnetic field. Many parameters, such as gas flow rates, the carbonyl generator temperature, and the gases used as the photosensitizer and sheath gas, can affect the nanoparticle production rate, size, and morphology.
example 3
Effect of Carbon Monoxide (CO) Flow Rate
[0047] After regenerating the nickel powder in the nickel carbonyl generator with flowing H2 at about 300° C., carbon monoxide (CO) can react with the active nickel surface to form nickel carbonyl at room temperature. The concentration and flow rate of Ni(CO)4 into the laser-driven reactor can be changed by varying the CO flow rate, and this, in turn, affects the production rate and the average size of the nickel nanoparticles. FIG. 3 shows the results that were obtained by fixing all other operating parameters and changing only the CO flow rate. The operating conditions are listed in Table 1. The average size obtained from XRD and BET experiments shown in FIG. 3 are different, since XRD estimates the average crystalline domain size, but nitrogen physisorption (the BET method) measures the specific surface area of the sample. From the surface area, the average particle size was calculated assuming that the particles are spheres with the densi...
PUM
Property | Measurement | Unit |
---|---|---|
diameter | aaaaa | aaaaa |
diameter | aaaaa | aaaaa |
diameter | aaaaa | aaaaa |
Abstract
Description
Claims
Application Information
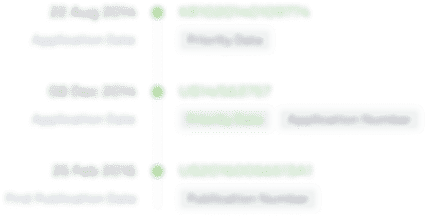
- R&D Engineer
- R&D Manager
- IP Professional
- Industry Leading Data Capabilities
- Powerful AI technology
- Patent DNA Extraction
Browse by: Latest US Patents, China's latest patents, Technical Efficacy Thesaurus, Application Domain, Technology Topic.
© 2024 PatSnap. All rights reserved.Legal|Privacy policy|Modern Slavery Act Transparency Statement|Sitemap