Drilling wellbores with optimal physical drill string conditions
- Summary
- Abstract
- Description
- Claims
- Application Information
AI Technical Summary
Benefits of technology
Problems solved by technology
Method used
Image
Examples
Embodiment Construction
[0028] Referring initially to FIG. 1, there is schematically illustrated a drilling system 10, which can be land-based or offshore, made according to one embodiment of the present invention for forming a wellbore 12 in a subterranean formation 14. The drilling system 10 includes a derrick 16 erected on a floor 18 that supports a rotary table 20 that is rotated by a prime mover. The system also includes a drill string 30 having a plurality of jointed tubulars 32 and a drilling assembly 40 coupled at an end thereof. The drilling assembly 40 is also referred to as a bottom hole assembly (BHA) 40. A control unit 50 controls drilling operations and a display device 52 provides the operator or driller information relating to drilling in pictorial, numeric, and alphanumeric formats. Other related components and equipment of the system 10 are well known in the art and is not described in detail herein. Also, it should be understood that applications other than rotary drives (e.g., coiled tu...
PUM
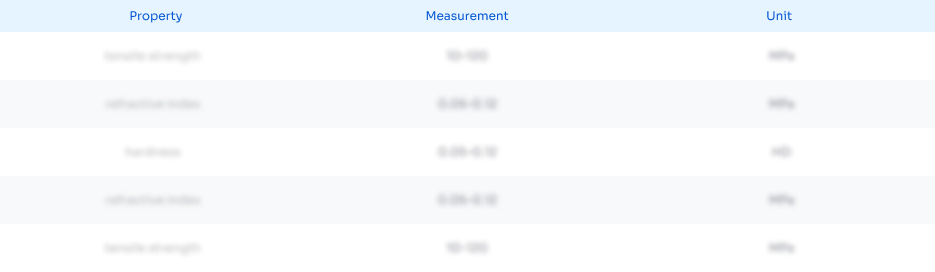
Abstract
Description
Claims
Application Information
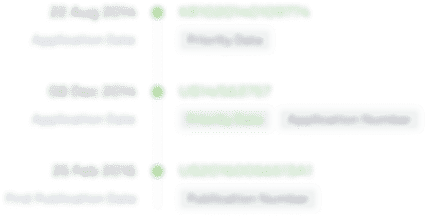
- R&D Engineer
- R&D Manager
- IP Professional
- Industry Leading Data Capabilities
- Powerful AI technology
- Patent DNA Extraction
Browse by: Latest US Patents, China's latest patents, Technical Efficacy Thesaurus, Application Domain, Technology Topic, Popular Technical Reports.
© 2024 PatSnap. All rights reserved.Legal|Privacy policy|Modern Slavery Act Transparency Statement|Sitemap|About US| Contact US: help@patsnap.com