Collector grid, electrode structures and interrconnect structures for photovoltaic arrays and methods of manufacture
a photovoltaic array and electrode structure technology, applied in the direction of basic electric elements, electrical equipment, semiconductor devices, etc., can solve the problems of thwarting the wide-scale energy collection of single-crystal silicon cells, and limiting the ability of the conductive surface to collect and transport current. , to achieve the effect of eliminating deficiencies
- Summary
- Abstract
- Description
- Claims
- Application Information
AI Technical Summary
Benefits of technology
Problems solved by technology
Method used
Image
Examples
process example (
B)
[0256]An alternative process is taught in conjunction with FIGS. 59 and 60. FIG. 59 is a top view of the process and FIG. 60 is a perspective view. The process is embodied in FIGS. 59 and 60 using the “tabbed cell stock”270 as shown in FIG. 55. One will recognize that other forms of “tabbed cell stock” such as those shown in FIGS. 23, 33, 42, are also suitable. A single strip of “tabbed” cell stock 270 is unwound from roll 300 and cut to a predetermined length “Y-59”. “Y-59” represents the width of the form factor of the eventual interconnected array. The strip of “tabbed cell stock” cut to length “Y-59” is then positioned. In the embodiment of FIGS. 59 and 60 the strip is securely positioned on vacuum belt 302. The strip is then “shuttled” in the original “x” direction of the “tabbed cell stock” a distance substantially the length of a repeat dimension among adjacent series connected cells. This repeat distance is indicated in FIGS. 56 and 59 as “X-10”. A second strip of “tabbed ...
example 1
[0261]A standard plastic laminating sheet from GBC Corp. 75 micrometer (0.003 inch) thick was coated with DER in a pattern of repetitive fingers joined along one end with a busslike structure resulting in an article as embodied in FIGS. 16 through 19. The fingers were 0.020 inch wide, 1.625 inch long and were repetitively separated by 0.150 inch. The buss-like structure which contacted the fingers extended in a direction perpendicular to the fingers as shown in FIG. 16. The buss-like structure had a width of 0.25 inch. Both the finger pattern and buss-like structure were printed simultaneously using the same DER ink and using silk screen printing. The DER printing pattern was applied to the laminating sheet surface formed by the sealing layer (i.e. that surface facing to the inside of the standard sealing pouch).
[0262]The finger / buss pattern thus produced on the lamination sheet was then electroplated with nickel in a standard Watts nickel bath at a current density of 50 amps. per s...
example 2
[0266]Individual thin film CIGS semiconductor cells comprising a stainless steel supporting substrate 0.001 inch thick were cut to dimensions of 7.5 inch length and 1.75 inch width.
[0267]In a separate operation, multiple laminating collector grids were prepared as follows. A 0.002 inch thick film of Surlyn material was applied to both sides of a 0.003 inch thick PET film to produce a starting laminating substrate as embodied in FIG. 44. Holes having a 0.125 inch diameter were punched through the laminate to produce a structure as in FIG. 48. A DER ink was then printed on opposite surfaces and through the holes to form a pattern of DER traces. The resulting structure resembled that depicted in FIG. 51. The grid fingers 254 depicted in FIGS. 50 and 51 were 0.012 inch wide and 1.625 inch long and were spaced on centers 0.120 inch apart in the length direction. The grid fingers 252 were 0.062 inch wide and extended 1 inch and were spaced on centers 0.5 inch apart. The printed film was t...
PUM
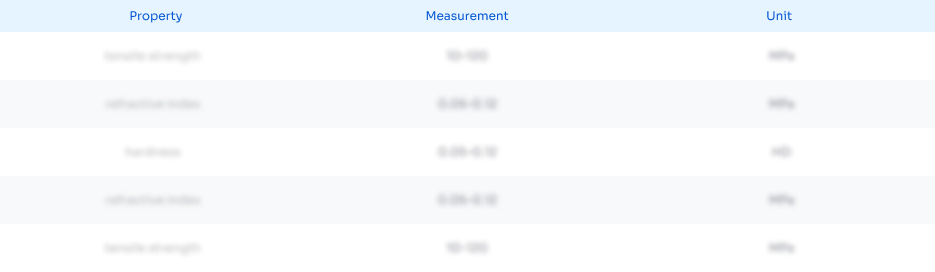
Abstract
Description
Claims
Application Information
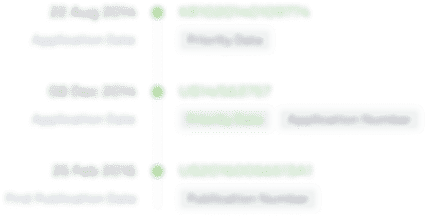
- Generate Ideas
- Intellectual Property
- Life Sciences
- Materials
- Tech Scout
- Unparalleled Data Quality
- Higher Quality Content
- 60% Fewer Hallucinations
Browse by: Latest US Patents, China's latest patents, Technical Efficacy Thesaurus, Application Domain, Technology Topic, Popular Technical Reports.
© 2025 PatSnap. All rights reserved.Legal|Privacy policy|Modern Slavery Act Transparency Statement|Sitemap|About US| Contact US: help@patsnap.com