Low Temperature Electrolytes for Solid Oxide Cells Having High Ionic Conductivity
- Summary
- Abstract
- Description
- Claims
- Application Information
AI Technical Summary
Benefits of technology
Problems solved by technology
Method used
Image
Examples
example 1
[0213]FIG. 3 depicts one embodiment of the invention in the form of a solid oxide cell having a metal oxide electrolyte 380 positioned between a first electrode 310 and a second electrode 320. The metal oxide electrolyte 380 comprises a powder 350 together with a metal oxide 360. In some cases, the powder 350 can be mixed with one or more metal compounds to form a slurry that is then applied by spin coating, brushing, or other suitable method onto the first electrode 310 (or second electrode 320). Then, the metal compound is converted to form the metal oxide 360, for example, by heating the atmosphere about the metal compound, or by inductively heating the first electrode 310. Optionally, once a layer of the metal oxide electrolyte 380 has been formed, additional powder-metal compound slurry can be applied and heated to form a thicker metal oxide electrolyte 380. The cell is assembled by placing the second electrode 320 onto the metal oxide electrolyte 380, or, optionally, additiona...
example 2
[0215]FIG. 4 depicts another embodiment of the present invention, in which a first metal oxide 450 and a second metal oxide 460, disposed in interpenetrating domains of metal oxides, form a metal oxide electrolyte between two electrodes 410, 420. To form such domains, a first metal compound composition is applied to the first electrode 410 and converted to a first metal oxide 450, such as, for example, strontium titanate. Then, a second metal compound composition is applied to the first metal oxide 450 and allowed to accumulate in pores, imperfections, and defects in the first metal oxide so formed. The second metal oxide composition is converted to form a second metal oxide 460, such as, for example, yttria-stabilized zirconia. Six alternating layers of the first metal oxide 450 and the second metal oxide 460 are formed in this embodiment.
[0216]In operation, for example, air or other oxygen-containing gas is supplied to the first electrode 410, which acts as the cathode to reduce d...
example 3
[0217]FIG. 5 depicts a solid oxide cell according to one embodiment of the present invention. A nanobar 540 and a metal oxide 560, disposed so that the nanobars 540 orient substantially perpendicularly to a first planar electrode 510, form a metal oxide electrolyte 580 between two electrodes 510, 520. The nanobar 540 can be, for example, a multi-walled carbon nanotube of semiconductor characteristic, oriented in metal oxide 560 which can be, for example, yttria-stabilized zirconia. To make the cell of FIG. 5, chosen nanobars 540 are combined with at least one metal compound in a metal compound composition, that is then applied to the first electrode 510. An orienting force is then applied. Optionally, the first electrode with the metal compound composition is placed in a magnetic field, at least a portion of the nanobars orient due to the magnetic field, and the metal compound composition is converted to form the metal oxide 560. Or, an electric field is applied to orient at least a...
PUM
Property | Measurement | Unit |
---|---|---|
Electrical conductivity | aaaaa | aaaaa |
Abstract
Description
Claims
Application Information
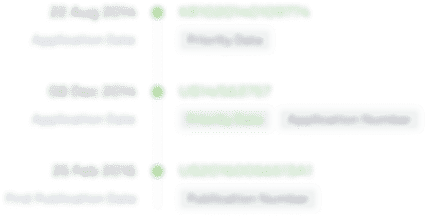
- R&D
- Intellectual Property
- Life Sciences
- Materials
- Tech Scout
- Unparalleled Data Quality
- Higher Quality Content
- 60% Fewer Hallucinations
Browse by: Latest US Patents, China's latest patents, Technical Efficacy Thesaurus, Application Domain, Technology Topic, Popular Technical Reports.
© 2025 PatSnap. All rights reserved.Legal|Privacy policy|Modern Slavery Act Transparency Statement|Sitemap|About US| Contact US: help@patsnap.com