Additive manufacturing with nanofunctionalized precursors
- Summary
- Abstract
- Description
- Claims
- Application Information
AI Technical Summary
Benefits of technology
Problems solved by technology
Method used
Image
Examples
examples
[0237]Materials. Aluminum alloy 7075 micropowder is purchased from Valimet Inc. (Stockton, Calif., U.S.). The powder consists of Al (balance), Zn (5.40%), Mg (2.25%), Cu (1.54%), Cr (0.19%), Fe (0.17%), Si (0.13%), Mn (0.02%), and Ti (2 powder) is purchased from US Research Nanomaterials Inc. (Houston, Tex., U.S.).
[0238]Selective Laser Melting. Additive manufacturing of stock aluminum alloy and functionalized aluminum alloy powders is performed on a Concept Laser M2 selective laser melting machine with single-mode, CW modulated ytterbium fiber laser (1070 nm, 400 W), scan speed up to 7.9 m / s, spot size 50 μm minimum. Powder handling parameters: 80 mm×80 mm build chamber size, 70 mm×70 mm build plate size, 20-80 μm layer thickness. The atmosphere is Ar or N2, 2. Samples consist of 60 mm×20 mm×40 mm tensile block specimens and 10 mm×10 mm×40 mm blocks for examining microstructure. Samples are processed with the Concept Laser ‘islanding’ scan strategy, which was specifically developed ...
PUM
Property | Measurement | Unit |
---|---|---|
Grain size | aaaaa | aaaaa |
Grain size | aaaaa | aaaaa |
Fraction | aaaaa | aaaaa |
Abstract
Description
Claims
Application Information
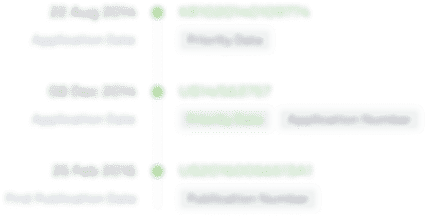
- R&D
- Intellectual Property
- Life Sciences
- Materials
- Tech Scout
- Unparalleled Data Quality
- Higher Quality Content
- 60% Fewer Hallucinations
Browse by: Latest US Patents, China's latest patents, Technical Efficacy Thesaurus, Application Domain, Technology Topic, Popular Technical Reports.
© 2025 PatSnap. All rights reserved.Legal|Privacy policy|Modern Slavery Act Transparency Statement|Sitemap|About US| Contact US: help@patsnap.com