EGR cooling and condensate regulation system for natural gas fired co-generation unit
a technology of condensate regulation and natural gas, which is applied in the direction of machines/engines, mechanical equipment, and non-fuel substance addition to fuel, etc., can solve the problems of not providing adequate incentives for industry entities to construct generating facilities, the failure of traditional energy producers to replace spent units and capitalize new plants, and the inability of alternative energy producers to achieve the effects of reducing nox emission, reducing unwanted condensation, and increasing efficiency of natural gas fired co/generation systems
- Summary
- Abstract
- Description
- Claims
- Application Information
AI Technical Summary
Benefits of technology
Problems solved by technology
Method used
Image
Examples
Embodiment Construction
[0047] In accordance with the instant invention, a natural gas fueled, internal combustion engine, employing exhaust gas recycle (EGR), delivers power to spin a coupled electric turbine, as well as heat of combustion, through a heat exchanger, to a co-generation process / utility heat loop for on site use as heat for process water, utility heat, space heat, potable hot water, and the like. This is accomplished with the instant system by increasing the transfer of engine heat to the co-generation process / utility heat loop while maintaining the engine, and especially the cylinder combustion temperature low enough to increase efficiency and reduce thermal NOx to acceptable levels, even in the presence of the recycled exhaust gas. This is accomplished with substantially no increase in parasitic power requirements, such as adding external pumps to increase the flow through the heat exchanger and decreased necessity for catalytic exhaust system clean up.
[0048] As air is inducted into the c...
PUM
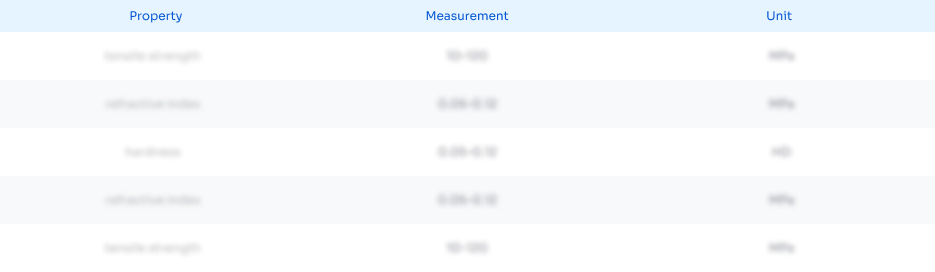
Abstract
Description
Claims
Application Information
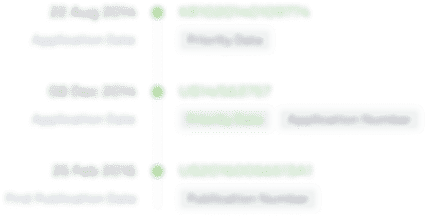
- R&D Engineer
- R&D Manager
- IP Professional
- Industry Leading Data Capabilities
- Powerful AI technology
- Patent DNA Extraction
Browse by: Latest US Patents, China's latest patents, Technical Efficacy Thesaurus, Application Domain, Technology Topic.
© 2024 PatSnap. All rights reserved.Legal|Privacy policy|Modern Slavery Act Transparency Statement|Sitemap