Dyes for analysis of protein aggregation
a protein aggregation and dye technology, applied in the field of dyes, can solve the problems of irreversible aggregation, protein aggregation mechanism, and eventual precipitation, and achieve the effects of reducing the number of dyes, and improving the quality of dyes
- Summary
- Abstract
- Description
- Claims
- Application Information
AI Technical Summary
Benefits of technology
Problems solved by technology
Method used
Image
Examples
example 1
Testing Compounds for Ability to Sense Protein Aggregation
[0225]Fluorescent readings were carried out in 50 mM Tris-HCl, pH 7.8 using 10 μM dye. When present, 1 μM recombinant human α-synuclein (ASN, Sigma-Aldrich, St. Louis, Mo.) as monomers, or aggregated as described in van Raaij et al. (2006) was included. Fluorescence excitation and emission spectra were collected on a Cary Eclipse fluorescence spectrophotometer (Varian, Australia). Fluorescence spectra were measured with excitation and emission slit widths set to 5 nm, and at a constant PMT voltage. Spectroscopic measurements were performed in standard quartz cells. All measurements were made at the respective excitation maxima of each dye. All measurements were carried out at room temperature. Results are summarized in Tables 1 and 2.
example 2
Fluorescence Sensitivity of Different Protein Aggregate-Sensing Dyes in the Presence of Excipients
[0226]IgG aggregate was prepared by adjusting 5.83 mg / ml of purified goat-anti-mouse IgG (H&L, Pel Freez, Rogers, Ark.) to pH 2.7 using HCl and incubating at 22° C. for 24 hours. The assay was performed using 2.8 μM IgG, either native or aggregated, and a dye concentration of 0.625 μM. The protein and dye were mixed together for 15 minutes at 22° C., then further incubated in the presence of the excipients shown in Table 5. The fluorescence intensity of S-25, Tol3 and Y2150 were determined with a FLUOstar OPTIMA plate reader (BMG LABTECH) at excitation wavelength of 550 nm and emission wavelength of 610 nm; while the fluorescence intensity for thioflavin-T was determined using a SpectraMAX GeminiXS (Molecular device, with Softmax Pro 7.0) using an excitation wavelength of 435 nm and emission wavelength of 495 nm. The fluorescence enhancement (aggregate / native IgG) is shown.
TABLE 5Effect...
example 3
Synthesis of S25
(a) Preparation of 6-methylsulfonyloxyhexyl methylsulfonate (Compound 1)
[0227]A solution of 1,6-hexanediol (13.15 g, 111.3 mmol) in 70 mL of anhydrous pyridine was cooled to 0° C. using ice bath. To this methanesulfonyl chloride (27 g, 235.7 mmol) was slowly added under mixing such that the temperature was maintained at 5-6° C. The combined mixture was stirred overnight at the temperature below 10° C. and the precipitate formed was filtered off, washed with 20% HCl (3×), water (3×), 5% solution of sodium bicarbonate (3×), and then again with water (3×). Product was dried under vacuum to obtain Compound 1 as a white solid (yield 32.8%). The structure of Compound 1 is given below:
(b) Preparation of Compound 2
[0228]A mixture of 4-methylpyridine (3.06 g, 32.9 mmol) and Compound 1 (4.11 g, 15 mmol) was heated at 120° C. for 3 hours. The reaction mixture was cooled and then 4 mL of isopropyl alcohol was added and the combined mixture was refluxed for an hour. After cooling...
PUM
Property | Measurement | Unit |
---|---|---|
temperatures | aaaaa | aaaaa |
time intervals | aaaaa | aaaaa |
temperature | aaaaa | aaaaa |
Abstract
Description
Claims
Application Information
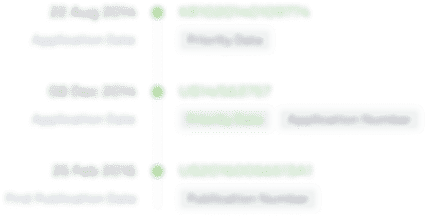
- R&D
- Intellectual Property
- Life Sciences
- Materials
- Tech Scout
- Unparalleled Data Quality
- Higher Quality Content
- 60% Fewer Hallucinations
Browse by: Latest US Patents, China's latest patents, Technical Efficacy Thesaurus, Application Domain, Technology Topic, Popular Technical Reports.
© 2025 PatSnap. All rights reserved.Legal|Privacy policy|Modern Slavery Act Transparency Statement|Sitemap|About US| Contact US: help@patsnap.com