Self-cohering, continuous filament non-woven webs
a non-woven web and continuous filament technology, applied in the field of self-cohering, continuous filament non-woven webs, can solve the problems of increasing the potential for colonization and wicking of bacteria within the interstices of aligned fiber bundles, high cost and complexity of knitting and weaving equipment, and few fibrous implants that utilize non-woven constructions
- Summary
- Abstract
- Description
- Claims
- Application Information
AI Technical Summary
Benefits of technology
Problems solved by technology
Method used
Image
Examples
example 1-- preparation
Example 1--Preparation of Web
164. 67% poly(glycolide) 33% poly(trimethylenecarbonate) (w / w) triblock copolymer was acquired from Davis & Geck (Danbury, Conn.)-Lot #10-CV-9433. This bioresorbable copolymer is commonly referred to by Davis & Geck as polyglyconate.
165. Approximately 25 mg of the acquired copolymer was dissolved in 25 ml of hexafluoroisopropanol (HFIP). The produced dilute solution was found to possess an inherent viscosity (IV) of 1.53 dl / g when measured using a Cannon-Ubelodde viscometer immersed in a 30.degree. C. (+ / -0.05.degree. C.) water bath.
166. Approximately 10 mg of the acquired copolymer was placed into an aluminum DSC sample pan, covered, and analyzed utilizing a Perkin-Elmer DSC 7 equipped with an Intracooler II cooling unit able to provide sample cooling to temperatures as low as -40.degree. C. After preconditioning of the sample at 180.degree. C. for 2 minutes, the sample was cooled at the maximum rate provided by the instrument (-500.degree. C. / min setti...
example 2--
Characterization of Example 1 Web
172. Filament Diameter
173. A sample of the cohesive web produced in Example 1 was observed utilizing both light microscopy and scanning electron microscopy (SEM) at magnifications between 20.times. and 1000.times. (see FIG. 2 for example). The examined web was found to be composed of fibers ranging in diameter from approximately 20 to 100 micrometers.
174. Web Bonding Characteristics
175. Further examination of the contact points between fibers utilizing both light microscopy and SEM at 1000.times. showed the web's fibers to physically intersect with each other with limited distortion or deformation of the contacting fibers' cylindrical form or nature. This observed physical intersection and interfiber contact was assumed to be autogenous self-cohesion since no adhesive binders or adjuncts had been added to the copolymer either before, during, or after the extrusion process described in Example 1.
176. No Noticeable Fraying
177. Visual examination also r...
example 3--
Shaping (Molding) of Web in 37.degree. C. Water
193. Arch Form
194. After over 24 months continuous storage under refrigerated conditions, a 2.5 cm.times.2.5 cm section was obtained from the web described in Example 1 and characterized in Example 2. The section of web was formed into an arch and restrained so that the outside edges of the arch were separated by approximately a 0.7 cm distance. This distance is intended to approximate the width of a mandibular ridge. The web section was then immersed into a 37.degree. C. water bath while maintaining its restrained arched configuration. After 10 minutes of 37.degree. C. immersion, the piece was removed from both the bath and its restraint and allowed to return to room temperature. The web was found to have retained its arched configuration upon removal.
195. The preformed arched web was then restrained so that the separation between the outer opposite edges was approximately 2.0 cm apart. The restrained web was then reimmersed into the 3...
PUM
Property | Measurement | Unit |
---|---|---|
Shear strength | aaaaa | aaaaa |
Shear strength | aaaaa | aaaaa |
Shear strength | aaaaa | aaaaa |
Abstract
Description
Claims
Application Information
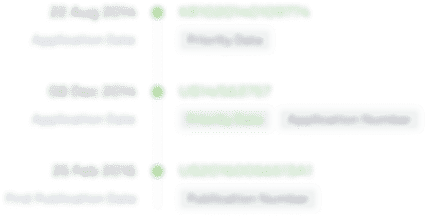
- R&D Engineer
- R&D Manager
- IP Professional
- Industry Leading Data Capabilities
- Powerful AI technology
- Patent DNA Extraction
Browse by: Latest US Patents, China's latest patents, Technical Efficacy Thesaurus, Application Domain, Technology Topic, Popular Technical Reports.
© 2024 PatSnap. All rights reserved.Legal|Privacy policy|Modern Slavery Act Transparency Statement|Sitemap|About US| Contact US: help@patsnap.com